Tracking the health of the nation through wastewater
Researchers at the University of Bath are developing a powerful, anonymous, real-time epidemiology tool.
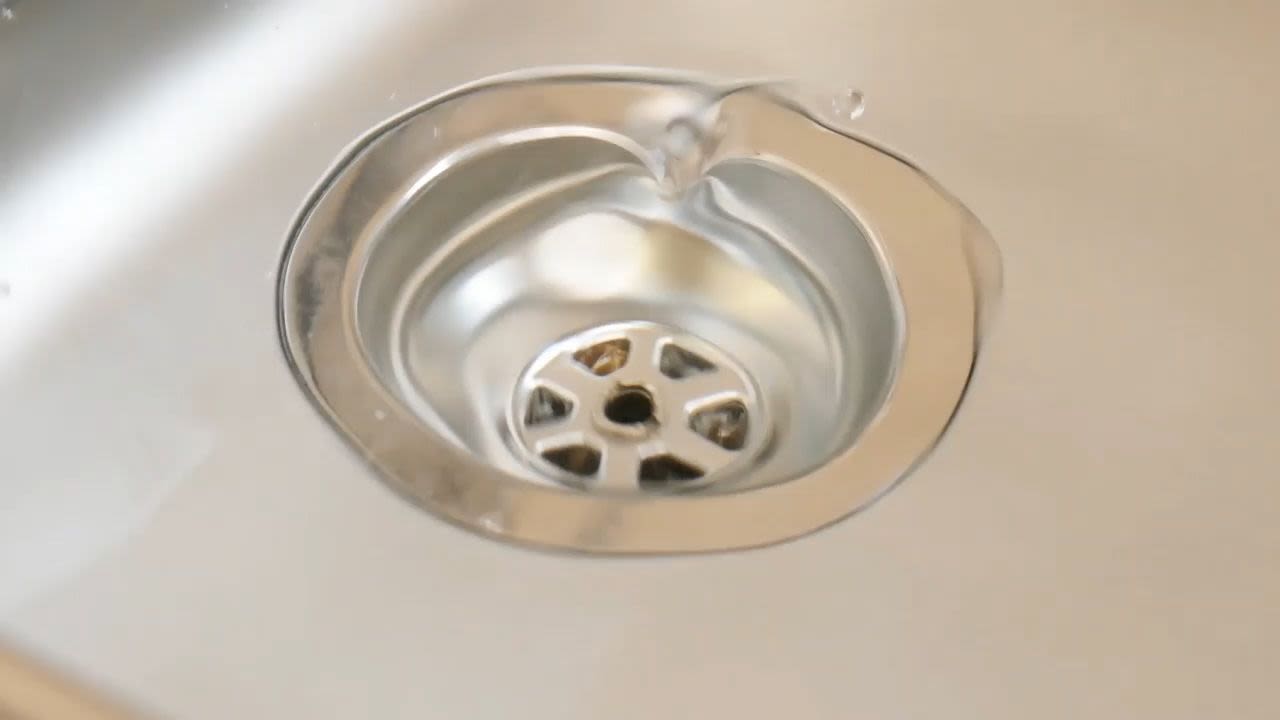
Imagine being able to take a snapshot of the health of a population without them having to fill out a single questionnaire or provide a urine sample. Where you can track health markers from a whole community, anonymously and in near real-time.
Professor Barbara Kasprzyk-Hordern and her colleagues from the Institute for Sustainability and the Water Innovation and Research Centre at the University of Bath have been developing an epidemiology tool that has the potential to do exactly this.
Over the last 15 years they have been working on wastewater-based epidemiology (WBE), a tool which analyses samples of wastewater from whole communities for 100+ chemicals that are either markers for health and disease, indicators of human exposure to hazardous chemicals or those that can adversely affect human health or the environment.
WBE is a powerful One Health approach that has the potential to be used to tackle a wide-ranging number of health issues, including tracking the spread of new epidemics, monitoring use of prescription medication or illicit drugs, measuring exposure to alcohol or tobacco, or even identifying which pesticides people have ingested via their food.
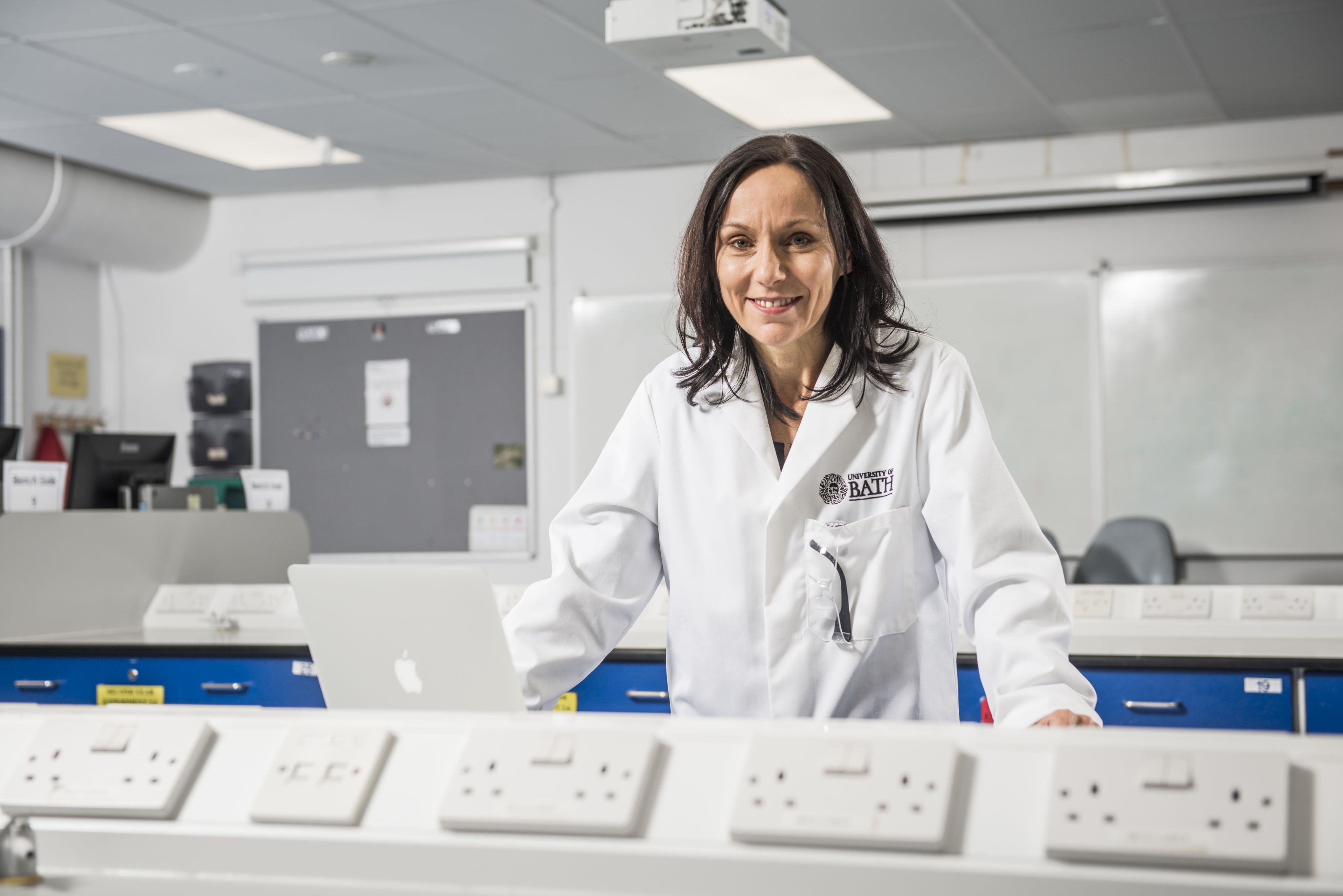
Professor Barbara Kasprzyk-Hordern
Professor Barbara Kasprzyk-Hordern
Prof Kasprzyk-Hordern says, “The trouble with monitoring public health using traditional epidemiology studies is that they rely on people filling out surveys or providing samples.
“Unfortunately, surveys have limitations in terms of accuracy of the data provided by individuals as well as the timescales required to collect such data. Also, the scope of such analyses is statistically limited on how many responses can be collected and it can be tricky to cover certain demographic groups that are traditionally difficult to recruit on these studies.
“Analysing wastewater is a bit like taking a pooled, very diluted, urine sample of a whole community. It therefore gives a fingerprint of that community’s collective health and lifestyle."
“The beauty of it is that the data is comprehensive, subjects are anonymous, and with enough resources, it can be collected and processed continuously and close to real-time.”
Powerful epidemiology tool
Work at Bath has made major contributions to developing this new technology, which is now widely used as an evidence-based public health prediction tool.
This research has already had an impact in increasing the understanding of illicit drug use across Europe, and in 2016 WBE was adopted by the European Monitoring Centre for Drugs and Drug Addiction (EMCDDA) to monitor trends of drug use and feed into their Europe-wide early warning system.
A long-standing research collaboration with local water supplier Wessex Water led to the establishment of the multi-partner Water Innovation and Research Centre at Bath (WIRC@Bath) in 2014.
This was followed in 2018 by the establishment of the GW4 Water Security Alliance, a partnership involving the water centres and institutes at the ‘GW4’ universities of Bath, Bristol, Cardiff and Exeter, which is the largest UK water research consortium and one of the largest worldwide.
As a result of working with Prof Kasprzyk-Hordern and her colleagues for more than a decade, Wessex Water has supported the development of Wastewater Based Epidemiology technologies to monitor public and environmental health risks including COVID-19 surveillance.
Wastewater profiling – a needle in a haystack
"Wastewater Based Epidemiology is conceptually simple, but methodologically very sophisticated."
Sample collection
Water samples are taken from rivers or wastewater treatment works.
How the water is sampled depends on the research question being investigated, but generally samples are taken from a location every 10-30 minutes over a 24 hour period, then pooled to give a composite sample for analysis.
Depending on the resources available and the scope of the study, samples can be taken daily, weekly or even over several years to identify trends.
Samples are cooled if they are being used for detecting biological markers such as DNA.
Internal standard controls are then added to maintain integrity of the sample.
Concentrating the sample
After filtering out solids particles, the chemicals of interest must be separated from the wastewater.
These markers are usually present in tiny trace amounts, meaning the majority of the sample must be removed.
The team does this by passing the sample through a column containing tiny polymer beads that have molecules attached which bind the specific chemicals being studied (analytes), whilst allowing the rest of the water to flow through.
Once the whole sample has passed through the column, the chemicals attached to the beads are removed, or eluted, using various solvents.
This effectively concentrates the sample from around 50-100 millilitres, down to around half a millilitre. This can then be analysed using Liquid Chromatography-Mass Spectrometry (LCMS).
Identifying the chemicals
The first stage, Liquid Chromatography, acts like a molecular sieve, further separating similar analytes by flowing the samples through silica beads that have been coated with molecules called aliphatic hydrocarbons.
These are carbon-based compounds, which interact differently with the chemicals in the sample, depending on their size, or chemical or physical properties.
Mass spectrometry
The sample then passes through a mass spectrometer, which evaporates the solvent from the liquid as it flows through, leaving the analyte molecules that are vapourised and ionised to become charged molecules.
The charged molecules are then separated according to their specific physical and chemical properties using electric and magnetic fields.
The machine can therefore identify each constituent chemical by its unique fingerprint, as well as quantify the amount present in the sample.
Analysing the data
Prof Kasprzyk-Hordern says, “Sometimes we do targeted analysis of a particular analyte, such as an antibiotic or drug molecule - we have developed methods that can analyse more than 100 targets simultaneously which saves time and resource and is critical for comprehensive biomarker profiling.
“Alternatively, we can collect full mass spectra followed by retrospective data mining and suspected or non-targeted screening to allow full water profiling.
“We have established a mass spectra repository at Bath to allow retrospective analysis. For example, we can work with historical data from 2015 and mass spectra collected in 2021 to understand the changing patterns of antimicrobial agents use and linked antimicrobial resistance.
“The technique is sensitive enough to detect traces of very small molecules such as pharmaceuticals, right up to large molecules like proteins. Whole bacteria or viruses can be also identified with mass spectrometry techniques.
“As well as detecting the chemicals themselves, we can also detect the biomarkers of exposure to these chemicals, so we can distinguish between chemicals that are present in the environment and which ones have passed through a human body.”
Linking chemicals with public health
This is particularly useful when estimating a population’s exposure to environmental contaminants such as household chemicals that might be linked with certain diseases such as cancer or diabetes, chemicals present in personal care products that are suspected of endocrine disruption activity or antimicrobial resistance, food contaminants such as pesticides, pathogenic organisms, and lifestyle chemicals including pharmaceuticals.
It can also be used as an early warning system to monitor the spread of infectious diseases and antibiotic resistance.
Mathematical modelling
One challenge is trying to calculate the number of people contributing to the sample.
To do this, the scientists look at a number of chemicals produced by the body, including ammonia (excreted through urine, but is also produced by the agricultural industry) and metabolites of chemicals like caffeine and nicotine.
These can be overlaid with catchment areas of the sewerage system and NHS patient lists, making adjustments to account for seasonal influxes of tourists and students in a city such as Bath.
Rainfall also dilutes the samples, so these need to be normalised to flow rates adjusted for the recent weather.
Prof Kasprzyk-Hordern works with University of Bath statisticians Prof Julian Faraway and Dr Theresa Smith to use mathematical modelling to account for these variables and process the huge amounts of data created by WBE.
Tracking illicit drugs usage across Europe
The story of Wastewater Based Epidemiology started around 15 years ago when Prof Kasprzyk-Hordern’s team began looking at illicit drugs that had found their way into the environment via the sewage system.
She says: “These drugs are obviously important because they can be toxic to aquatic species, and are being taken in large enough quantities for us to be able to see them in wastewater and in the receiving environment.
“It was Christian Daughton from US EPA who first made the link. He realised that these drugs are usually metabolised when they’ve gone through a human body.
"So, by detecting the metabolites, we can work backwards from each water sample to calculate usage in a particular community.”
In 2010, collaborating organisations across Europe created a network called Sewage Analysis CORe group Europe (SCORE), to build larger data sets to source issues they thought important.
Six countries took part initially, with the UK working in collaboration with Norway, the Netherlands, Italy, Spain and Switzerland.
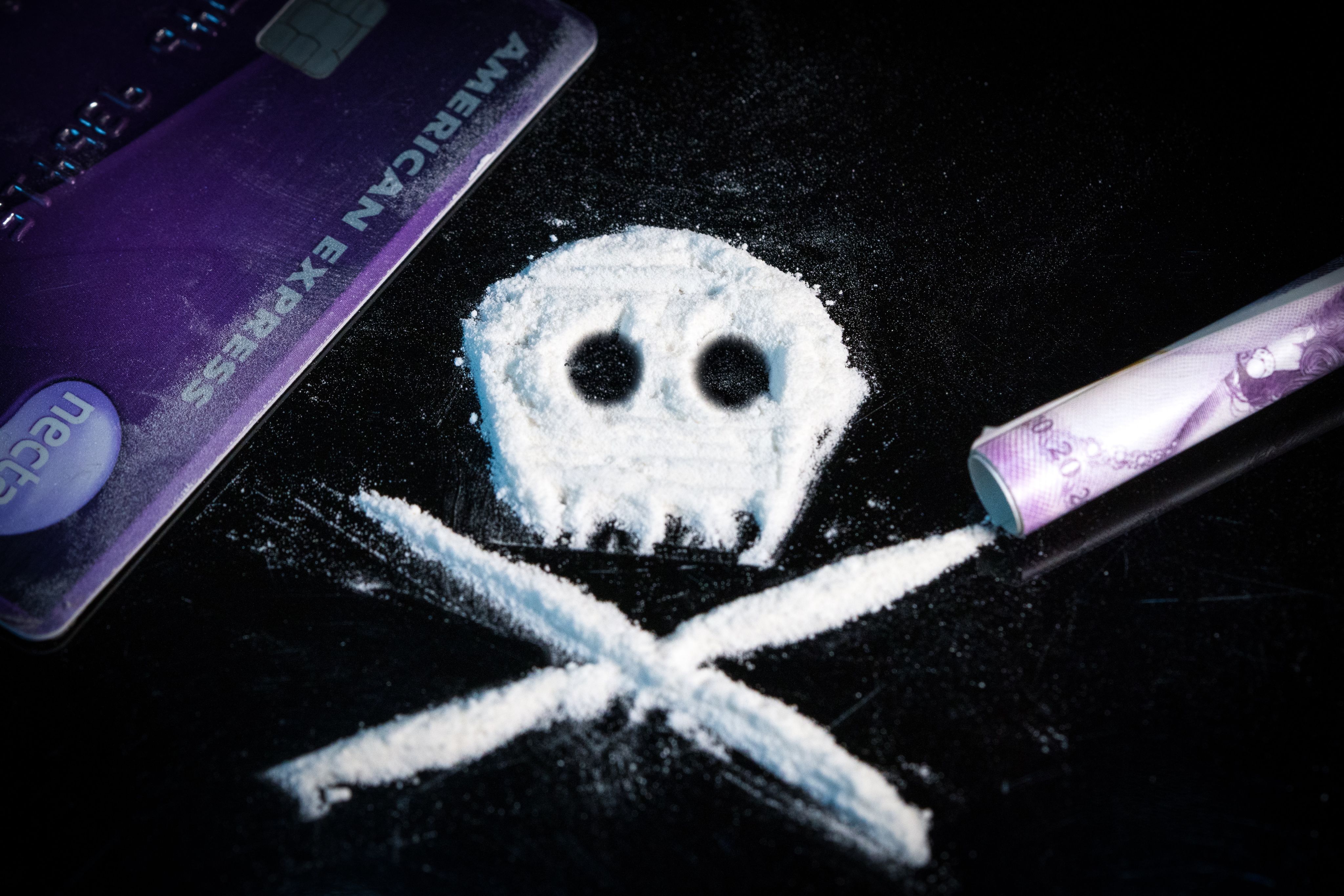
Photo by Colin Davis on Unsplash
Photo by Colin Davis on Unsplash
The SCORE group shared the vast amounts of data obtained with the European Monitoring Centre for Drugs and Drug Addiction (EMCDDA), which looks at trends and patterns of drugs, addiction and usage across the EU.
The team decided to investigate spatial-temporal connections, using funds to help with drug abuse.
Analysing wastewater in 19 European cities and towns, they were able to explore the drug-taking habits of people living there. Their results provided a remarkable snapshot of the illicit drug usage in these cities, with marked variations observed across different geographical locations.
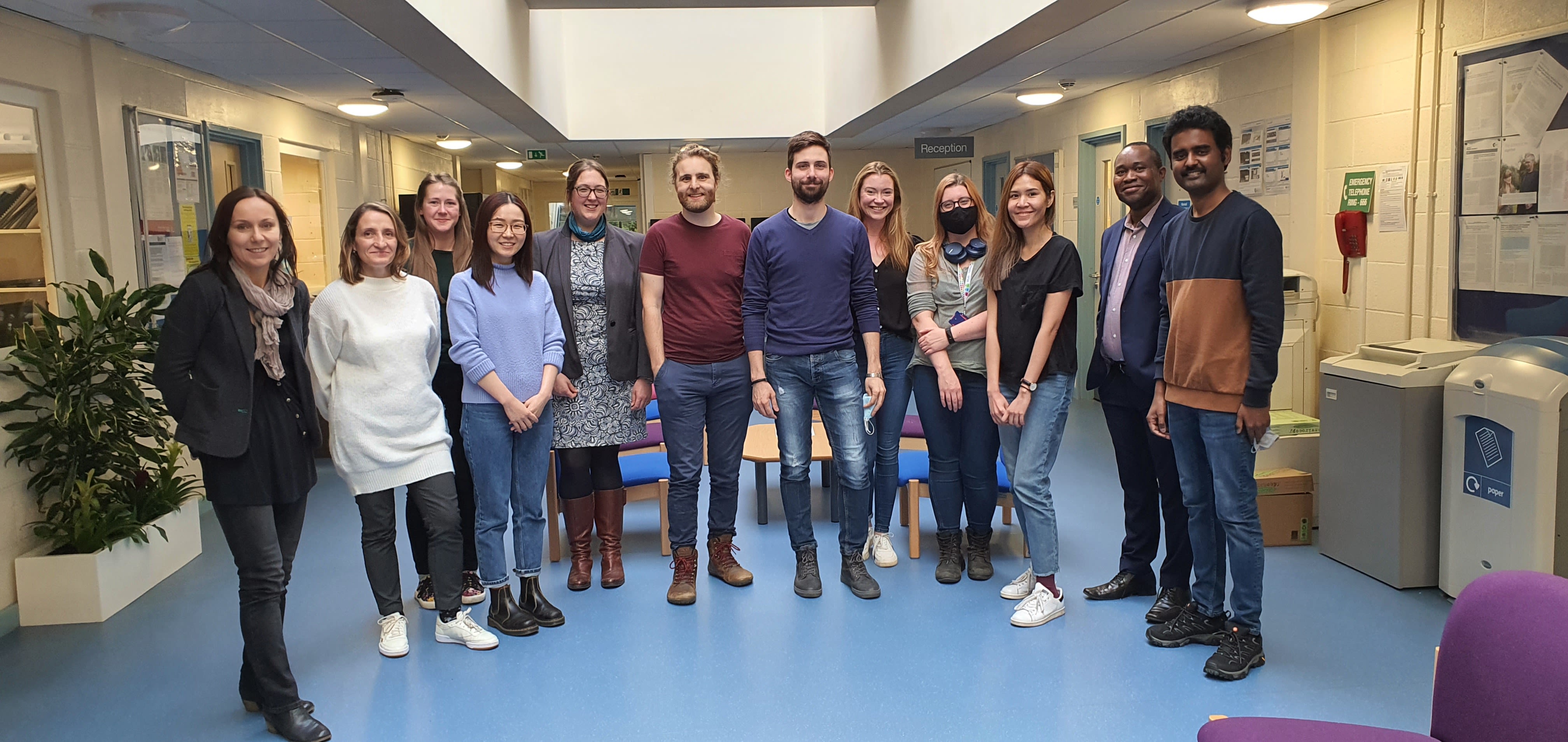
The Environmental Chemistry and Public Health Research Group at Bath
The Environmental Chemistry and Public Health Research Group at Bath
Following the success of this initial study, subsequent studies have included 75 cities across 25 countries, using a standard protocol allowing the researchers to directly compare illicit drug loads in Europe over a one-week period during 10 consecutive years.
Collecting 24 hour composite samples during a single week, the researchers tracked the “parent drug” molecules of amphetamine, methamphetamine and MDMA. The samples were also analysed for the main metabolites (molecules produced when the body breaks drugs down) of cocaine and cannabis.
Prof Kasprzyk-Hordern says: “Our techniques could also tell you where the chemicals we found were made, and which batch they’re from, as well as whether they had passed through a human body or flushed directly down the toilet during a police drugs raid.
“We could also identify when new drugs came on the black market, and alert the relevant public health bodies.”
Drug usage across Europe in 2021
The highest levels of cocaine use in 2021 were found in Amsterdam and Antwerp.
Amphetamine was higher in the north and east of Europe in 2021
Methamphetamine was generally lower than amphetamine but mainly concentrated in Czechia and Slovakia.
The highest amounts of MDMA were found in wastewater from cities in Belgium, Germany, the Netherlands, Sweden and Norway.
Cannabis was found in the highest concentrations in Croatia, Spain, the Netherlands and Slovenia (no data was collected from the UK).
The work of the SCORE group has led to the EMCDDA establishing an early warning system based on WBE, and when a new drug is found they send information on it to their headquarters in Lisbon.
This was the first practical application of the team’s data from WBE, however it was the outbreak of a novel coronavirus in 2020 that really sparked wider interest in the potential of this technology as a public health tool.
Tracking the COVID-19 epidemic in real time
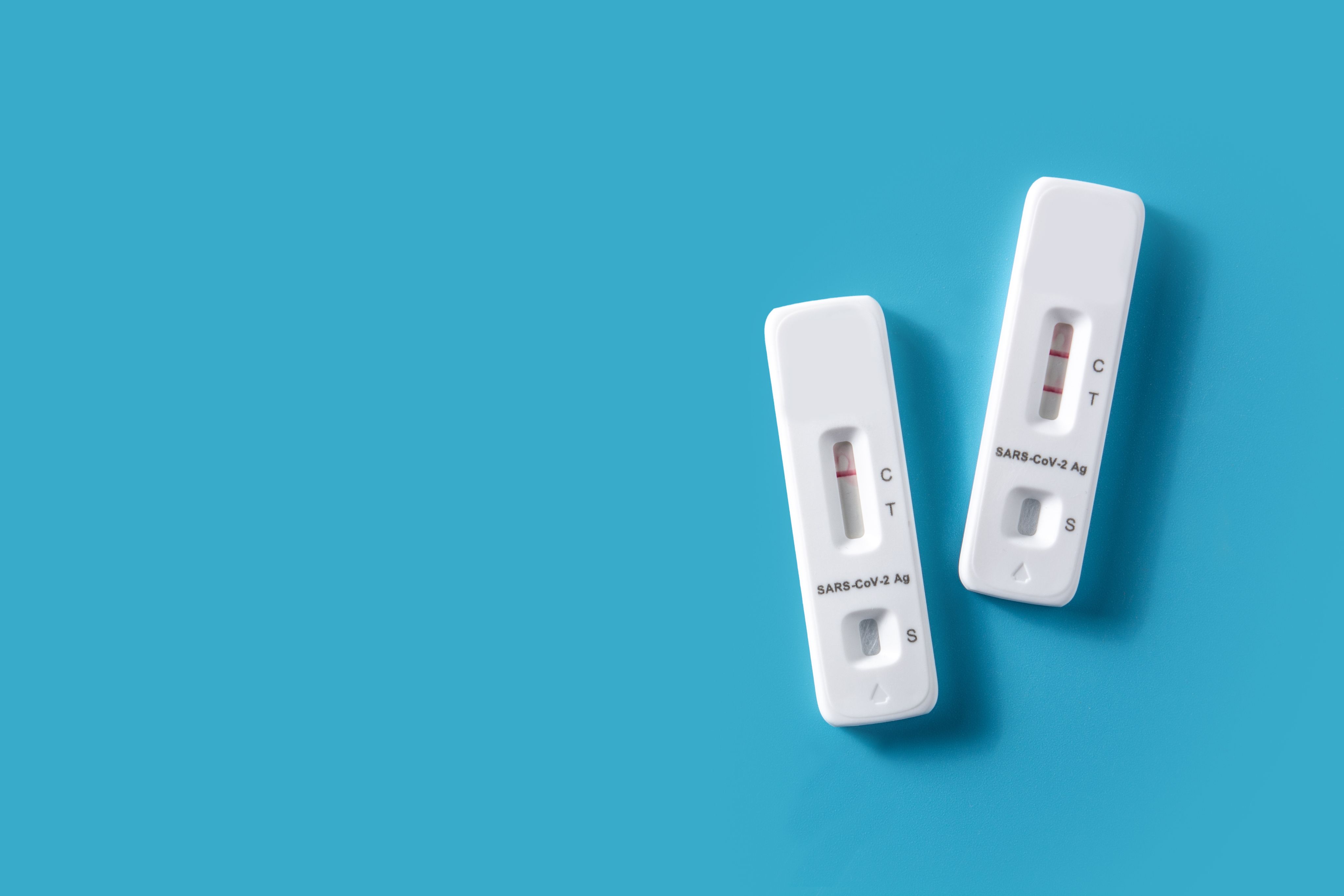
When the COVID-19 pandemic struck, it became apparent early on that getting data on community infection quickly was vital in making decisions on how to control the spread of the disease.
Prof Kasprzyk-Hordern and her colleagues immediately set about employing WBE to detect local outbreaks of SARS-CoV-2 infection (the virus that causes COVID-19) in the local area.
She says: “Scientists had already been investigating WBE as a surveillance tool for pathogens such as polio and enterovirus, so when COVID-19 emerged, we began work on detecting viral fragments of this new disease to track the incidence of COVID-19 in the local population.”
Prof Kasprzyk-Hordern’s team in the University of Bath’s Centre for Sustainable and Circular Technologies, together with Wessex Water, started monitoring the early days of the COVID-19 pandemic in March 2020, before the first lockdown in 2020 ironically paused their research for a few months.
Taking daily average wastewater samples from four locations in the Bristol-Bath region twice a week, they extracted the genetic material – in the case of SARS-CoV-2, RNA rather than DNA – and use a method called RT-qPCR to quantify the amounts of COVID-19 RNA present in the sample.
The team uses probes that are specific to genes that code for the proteins in the outer shell of the virus as well as the proteins inside the nucleus.
The technique can detect trace amounts of the virus, down to one gene per ml of wastewater.
James Boxall-Clasby, a PhD student in Integrated Sustainable Chemical Technologies working in the team says: “The exciting thing about this method is that it’s so sensitive, we can detect COVID-19 infections in the wastewater before people even realise they have the virus.
“So at the start of the pandemic, before routine home testing was taking place, we could estimate the levels of COVID-19 in the community in almost real time, even when carriers were symptomless or pre-symptomatic and so were not coming forward for testing.”
However the technique only measures levels of the virus in the community, which does not directly correlate to the number of people infected.
James explains: “We can’t accurately calculate the number of cases in a community from wastewater because it’s difficult to know variability in the amount of viral RNA shed per person – this will depend on what stage they are in their infection.
"One person who is heavily infected may be shedding the same number of virus particles as several asymptomatic people combined.
“However changes in signal can still provide useful data to public health teams - whereas Government data tells you how many people have tested positive that day, people tend to still be shedding virus for several days afterwards, so WBE can tell you how much virus is in the population in real time.
“Wastewater surveillance can also be used to non-invasively screen those communities that are traditionally difficult to test, whether due to low uptake of testing or lack of resources.”
Used strategically, WBE could be used as an early warning system to flag where further testing might be needed, or where a new outbreak might be occurring.
This is especially important now that free lateral flow testing has ended and few asymptomatic people are regularly testing for the virus.
It could be used for monitoring outbreaks in places such as schools, care homes and prisons, and could alert hospitals to prepare for incoming patients, for example by ordering PPE or freeing up intensive care beds.
“With WBE we can sample a much bigger proportion of the population rather than waiting for people to turn up and get tested.”
WBE data could also be used to investigate the effects of different policies, such as imposing curfews or local lockdowns versus national lockdowns, and for tracking the progress of vaccination campaigns in individual communities.
If WBE is employed routinely at every treatment works, researchers could potentially track seasonal norovirus or flu, identify local hotspots, as well as identify new pathogens as in the case of COVID-19.
The team also tested for other markers during COVID pandemics.
Prof Kasprzyk-Hordern’s PhD student in the University of Bath’s Centre for Sustainable and Circular Technologies, Nicola Ceolotto, contributed to the study by looking at various chemical markers including those needed to understand pain treatment in communities.
He says: “Testing for chemical markers alongside biological markers revealed changes in pain medication usage, including increased usage of certain anti-inflammatories, in and out of lockdown, as well as during the intensive vaccination period.
“We observed changes in lifestyle chemicals usage too, as well as chemicals used in disinfection products. These are important from an environmental health perspective as they can be toxic.”
Prof Kasprzyk-Hordern says: “We’ve shown this tool has big potential for tracking epidemics as well as understanding the bigger picture in terms of changes in behaviours and increased need for certain medications, however we need to collaborate further with public health practitioners to design sampling strategies in terms of location and frequency of sampling to make sure public health teams can access the data they need.”
The researchers are working collaboratively with other universities and public health bodies including the UK Health Security Agency (UKHSA) to develop an effective wastewater surveillance system for all biochemical markers, as well as viruses including COVID-19 and norovirus.
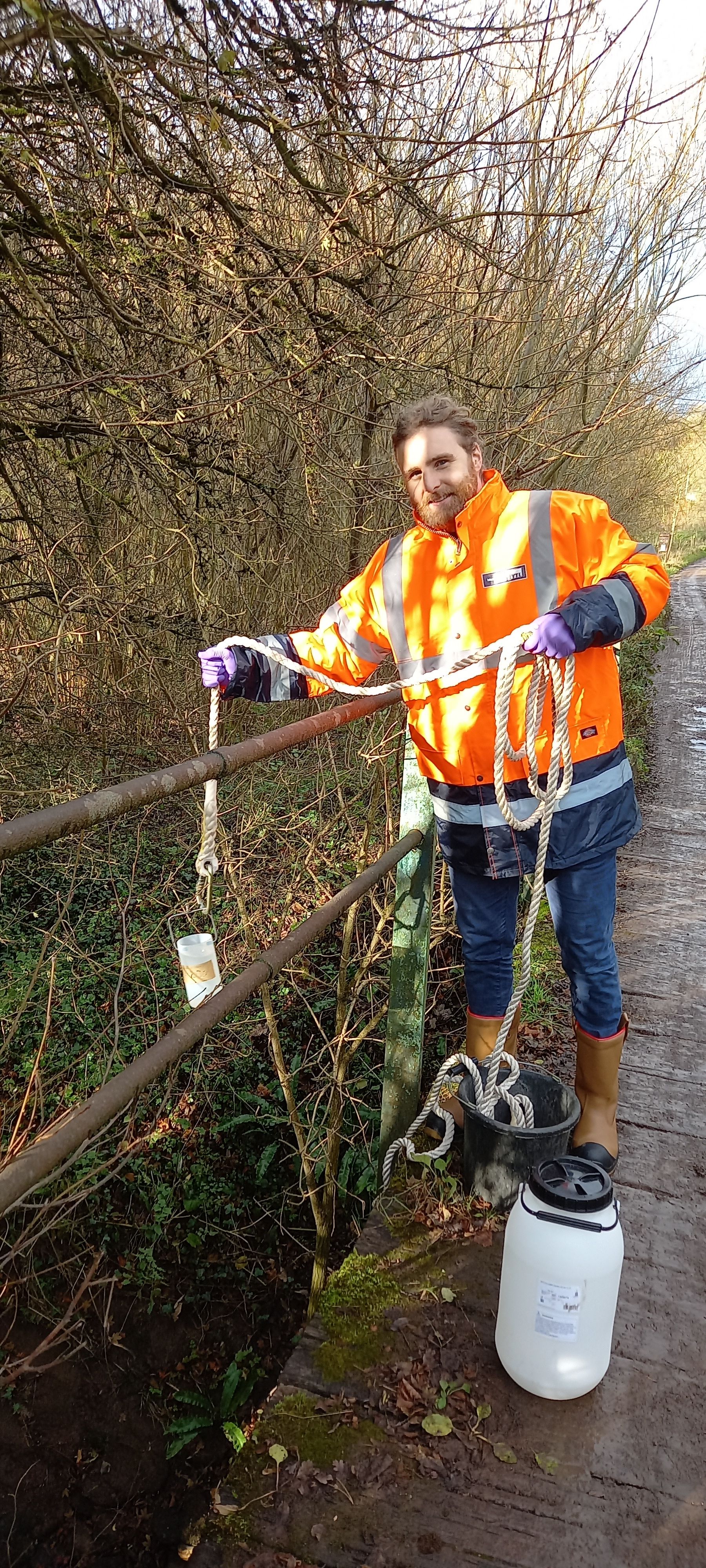
James Boxall-Clasby collecting a river sample for analysis
James Boxall-Clasby collecting a river sample for analysis
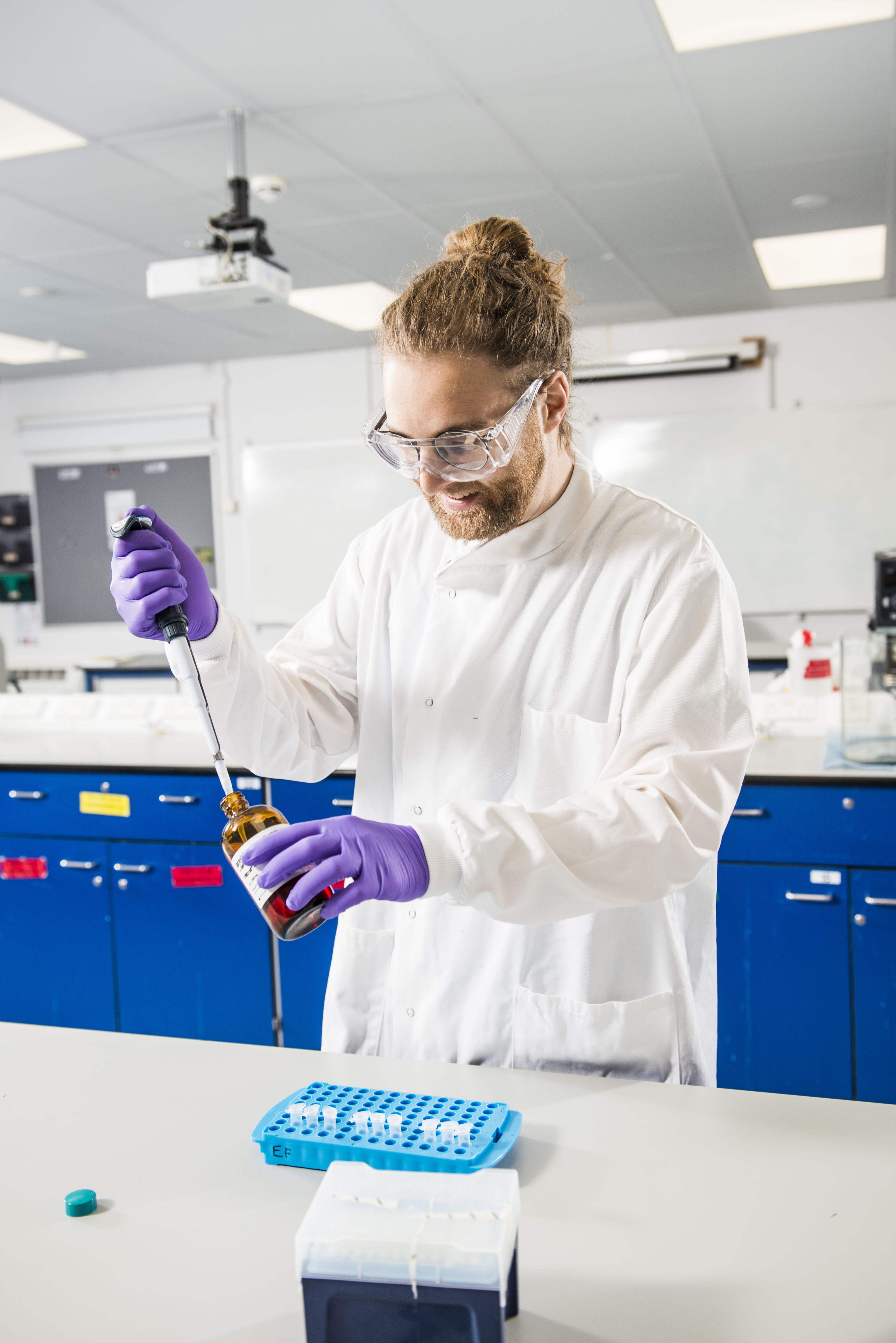
James preparing samples for PCR analysis
James preparing samples for PCR analysis
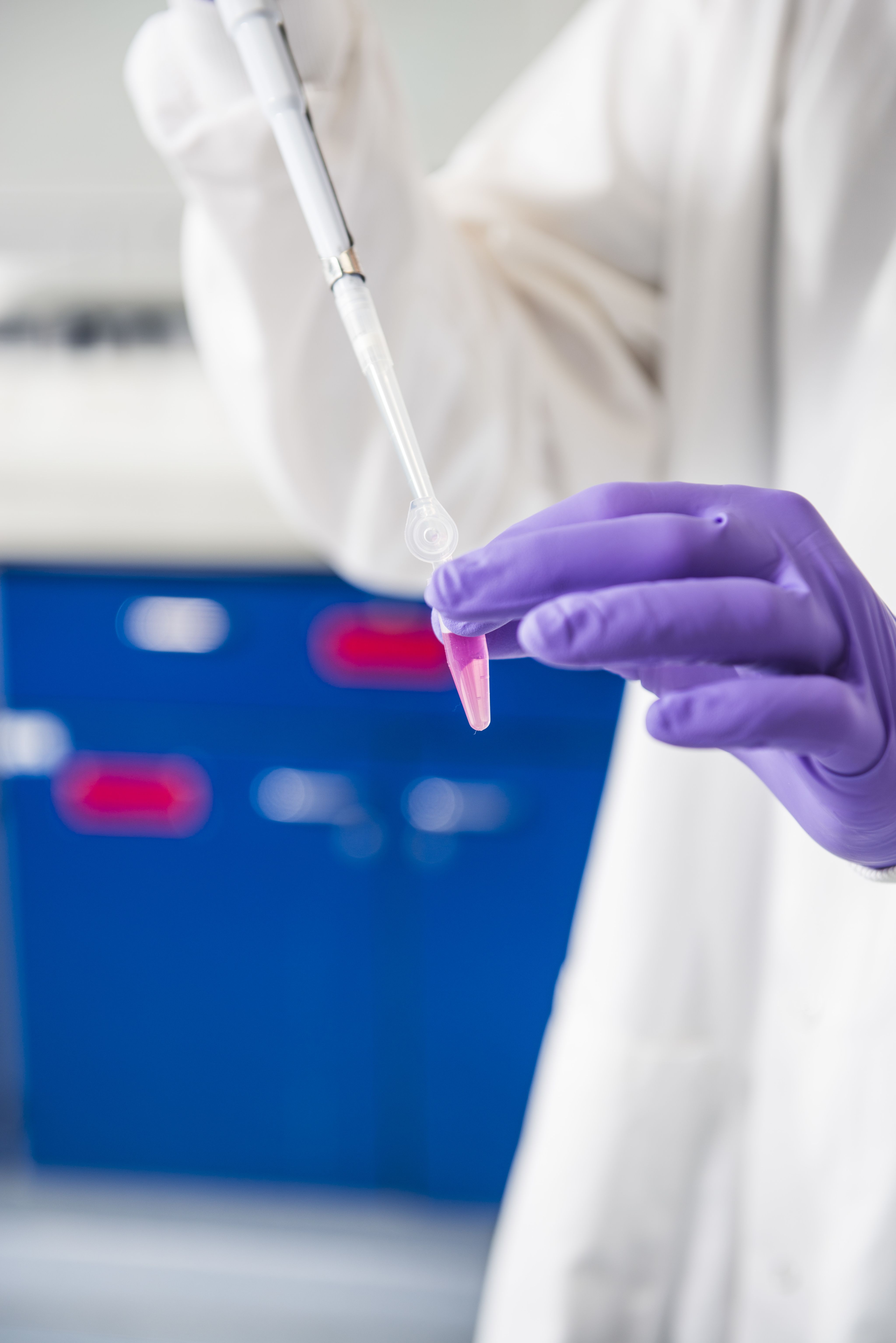
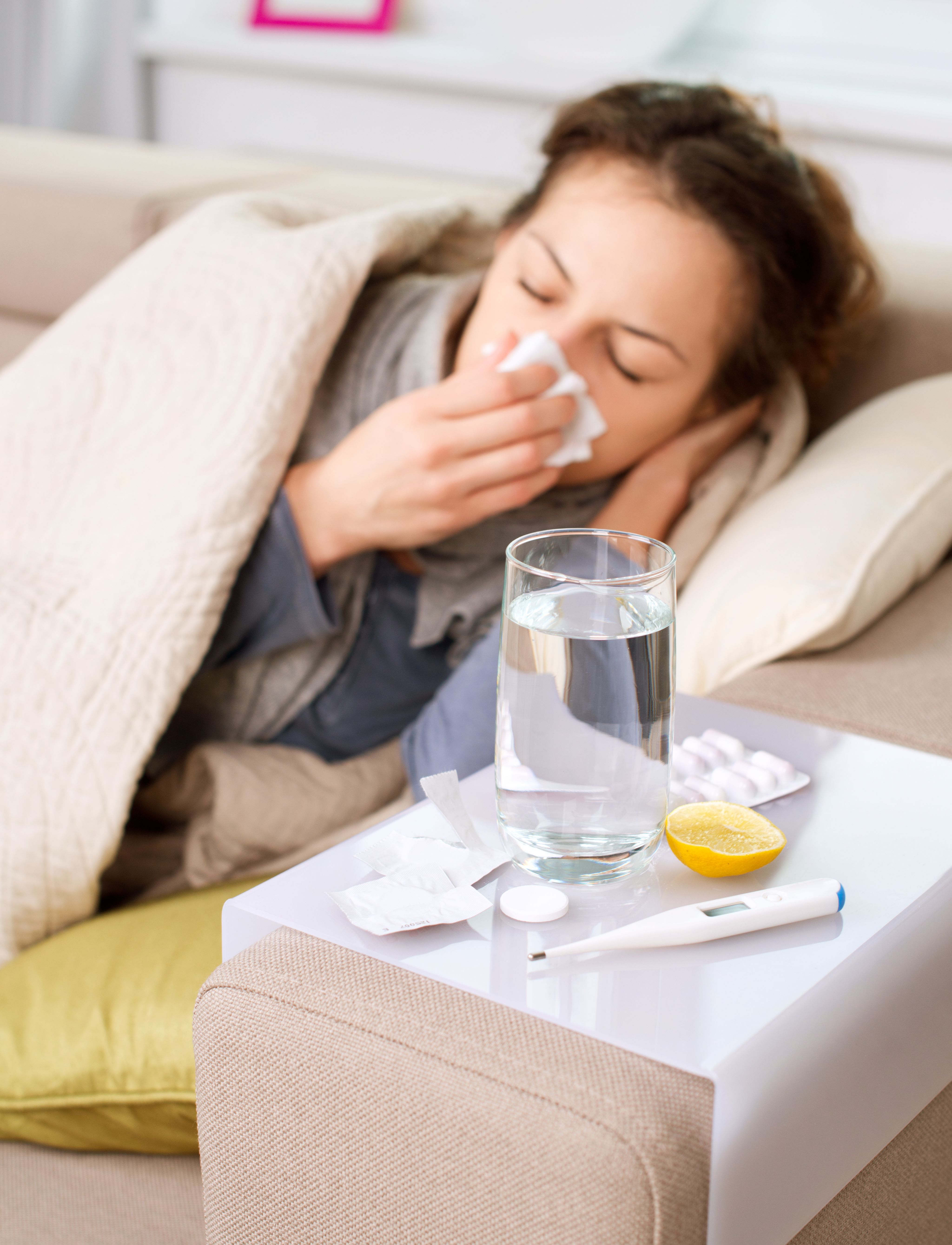
The team observed an increase in the use of pain medication during the peaks of the COVID-19 pandemic. (Credit: Subbotina Anna on Adobe Stock)
The team observed an increase in the use of pain medication during the peaks of the COVID-19 pandemic. (Credit: Subbotina Anna on Adobe Stock)
When the COVID-19 pandemic struck, it became apparent early on that getting data on community infection quickly was vital in making decisions on how to control the spread of the disease.
Prof Kasprzyk-Hordern and her colleagues immediately set about employing WBE to detect local outbreaks of SARS-CoV-2 infection (the virus that causes COVID-19) in the local area.
She says: “Scientists had already been investigating WBE as a surveillance tool for pathogens such as polio and enterovirus, so when COVID-19 emerged, we began work on detecting viral fragments of this new disease to track the incidence of COVID-19 in the local population.”
Prof Kasprzyk-Hordern’s team in the University of Bath’s Centre for Sustainable and Circular Technologies, together with Wessex Water, started monitoring the early days of the COVID-19 pandemic in March 2020, before the first lockdown in 2020 ironically paused their research for a few months.
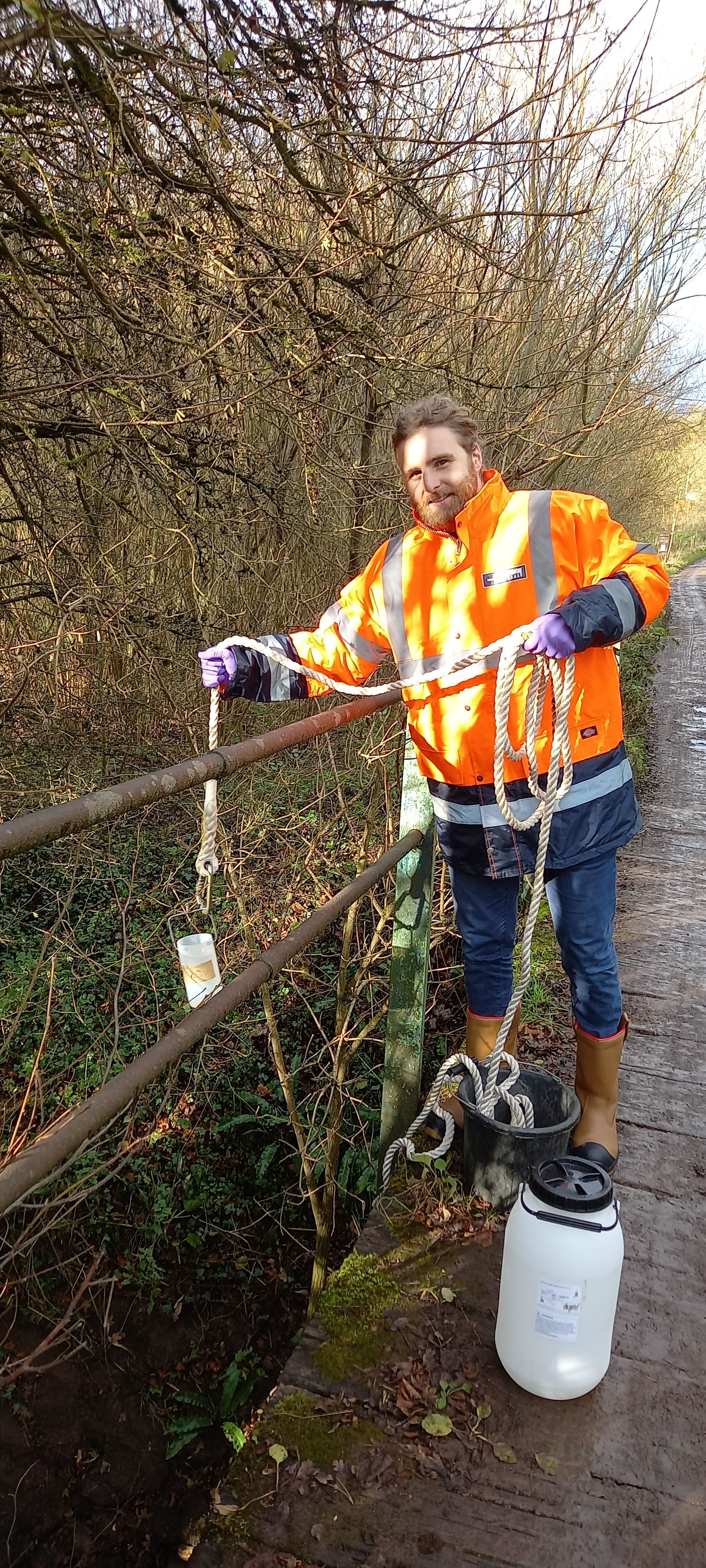
James Boxall-Clasby collecting a river sample for analysis
James Boxall-Clasby collecting a river sample for analysis
Taking daily average wastewater samples from four locations in the Bristol-Bath region twice a week, they extracted the genetic material – in the case of SARS-CoV-2, RNA rather than DNA – and use a method called RT-qPCR to quantify the amounts of COVID-19 RNA present in the sample.
The team uses probes that are specific to genes that code for the proteins in the outer shell of the virus as well as the proteins inside the nucleus.
The technique can detect trace amounts of the virus, down to one gene per ml of wastewater.
James Boxall-Clasby, a PhD student in Integrated Sustainable Chemical Technologies working in the team says: “The exciting thing about this method is that it’s so sensitive, we can detect COVID-19 infections in the wastewater before people even realise they have the virus.
“So at the start of the pandemic, before routine home testing was taking place, we could estimate the levels of COVID-19 in the community in almost real time, even when carriers were symptomless or pre-symptomatic and so were not coming forward for testing.”
However the technique only measures levels of the virus in the community, which does not directly correlate to the number of people infected.
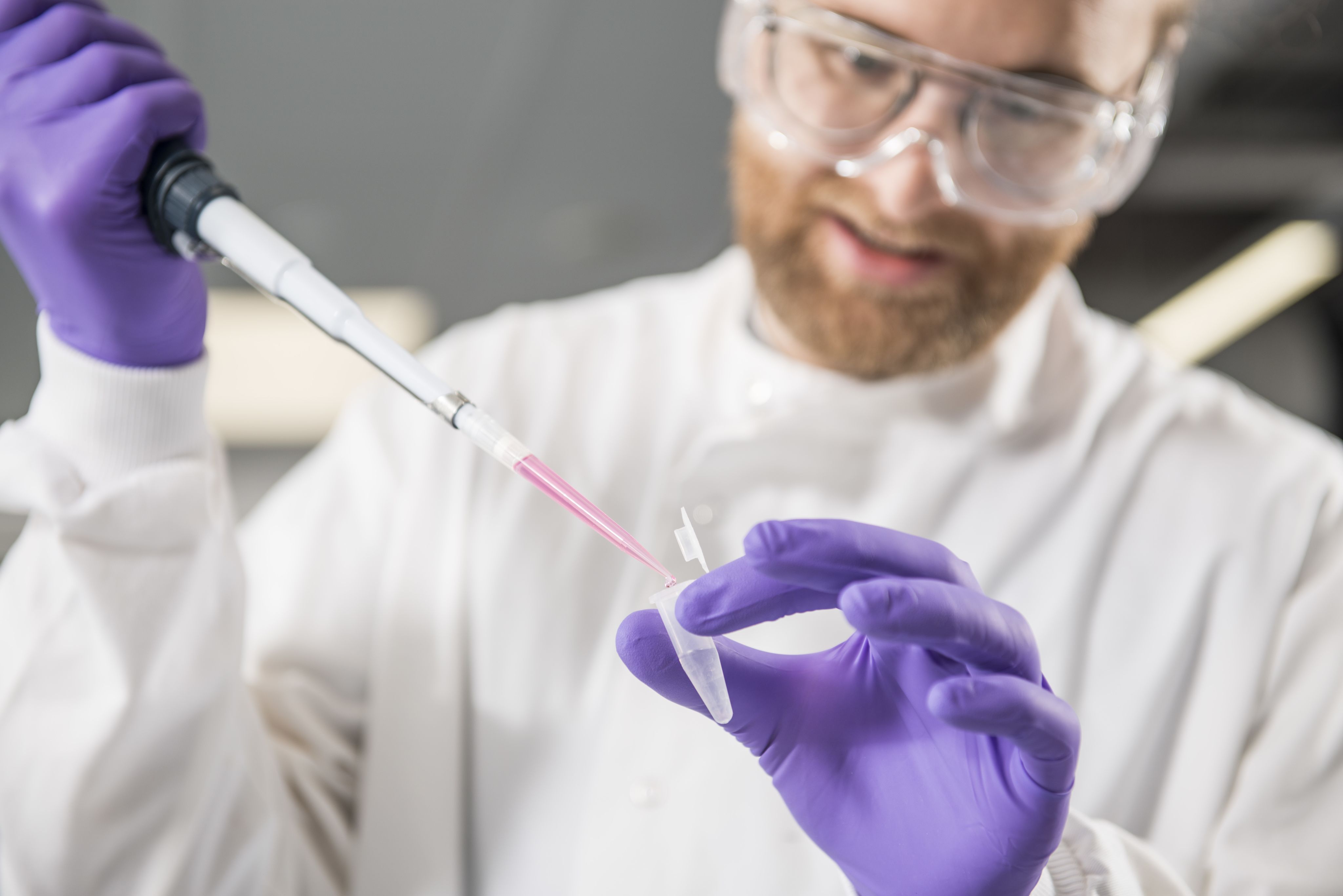
James preparing samples for PCR analysis
James preparing samples for PCR analysis
James explains: “We can’t accurately calculate the number of cases in a community from wastewater because it’s difficult to know variability in the amount of viral RNA shed per person – this will depend on what stage they are in their infection.
"One person who is heavily infected may be shedding the same number of virus particles as several asymptomatic people combined.
“However changes in signal can still provide useful data to public health teams - whereas Government data tells you how many people have tested positive that day, people tend to still be shedding virus for several days afterwards, so WBE can tell you how much virus is in the population in real time.
“Wastewater surveillance can also be used to non-invasively screen those communities that are traditionally difficult to test, whether due to low uptake of testing or lack of resources.”
Used strategically, WBE could be used as an early warning system to flag where further testing might be needed, or where a new outbreak might be occurring.
This is especially important now that free lateral flow testing has ended and few asymptomatic people are regularly testing for the virus.
It could be used for monitoring outbreaks in places such as schools, care homes and prisons, and could alert hospitals to prepare for incoming patients, for example by ordering PPE or freeing up intensive care beds.
“With WBE we can sample a much bigger proportion of the population rather than waiting for people to turn up and get tested.”
WBE data could also be used to investigate the effects of different policies, such as imposing curfews or local lockdowns versus national lockdowns, and for tracking the progress of vaccination campaigns in individual communities.
If WBE is employed routinely at every treatment works, researchers could potentially track seasonal norovirus or flu, identify local hotspots, as well as identify new pathogens as in the case of COVID-19.
The team also tested for other markers during COVID pandemics.
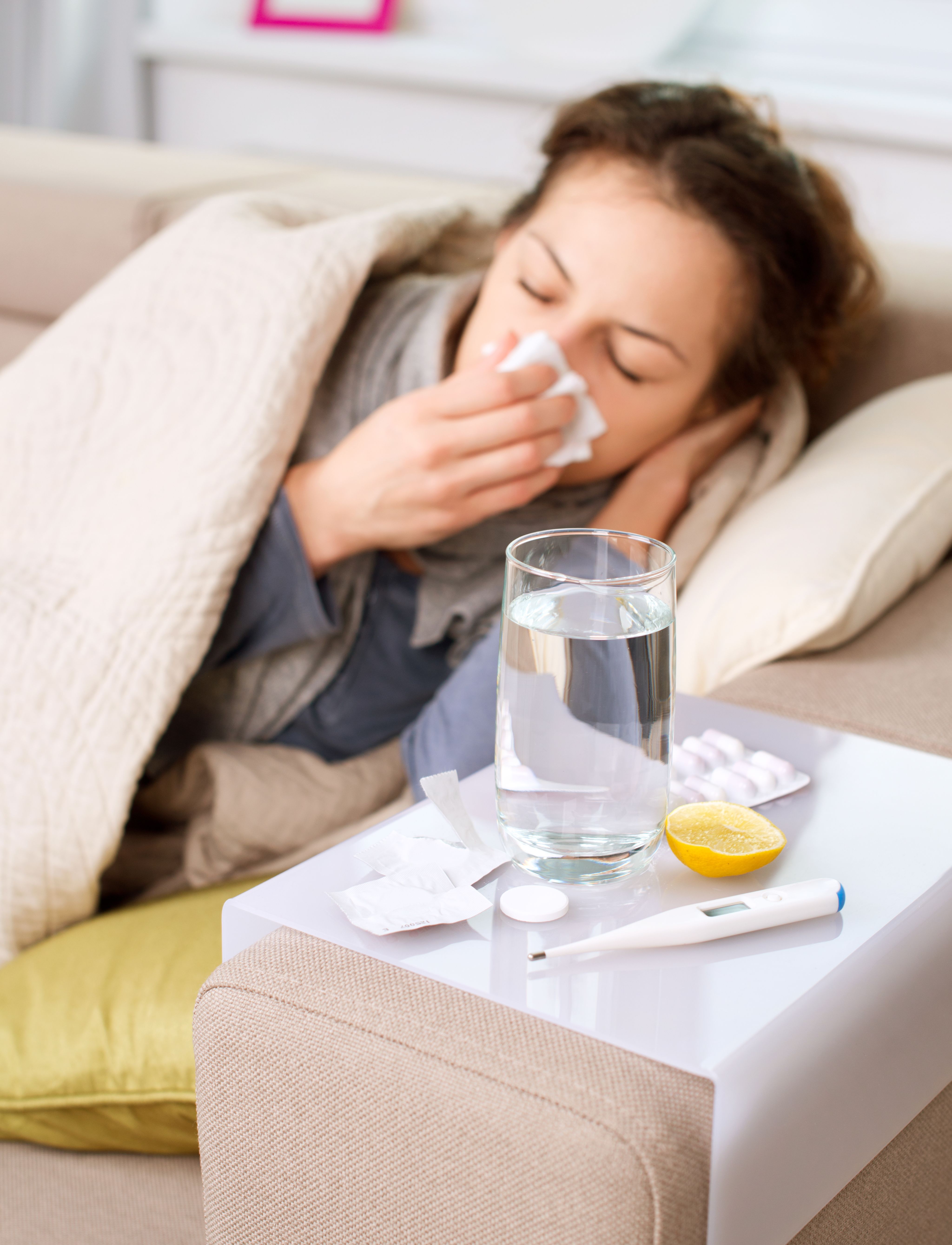
The team observed an increase in the use of pain medication during the peaks of the COVID-19 pandemic. (Credit: Subbotina Anna on Adobe Stock)
The team observed an increase in the use of pain medication during the peaks of the COVID-19 pandemic. (Credit: Subbotina Anna on Adobe Stock)
Prof Kasprzyk-Hordern’s PhD student in the University of Bath’s Centre for Sustainable and Circular Technologies, Nicola Ceolotto, contributed to the study by looking at various chemical markers including those needed to understand pain treatment in communities.
He says: “Testing for chemical markers alongside biological markers revealed changes in pain medication usage, including increased usage of certain anti-inflammatories, in and out of lockdown, as well as during the intensive vaccination period.
“We observed changes in lifestyle chemicals usage too, as well as chemicals used in disinfection products. These are important from an environmental health perspective as they can be toxic.”
Prof Kasprzyk-Hordern says: “We’ve shown this tool has big potential for tracking epidemics as well as understanding the bigger picture in terms of changes in behaviours and increased need for certain medications, however we need to collaborate further with public health practitioners to design sampling strategies in terms of location and frequency of sampling to make sure public health teams can access the data they need.”
The researchers are working collaboratively with other universities and public health bodies including the UK Health Security Agency (UKHSA) to develop an effective wastewater surveillance system for all biochemical markers, as well as viruses including COVID-19 and norovirus.
Public health multi-hazard early warning system
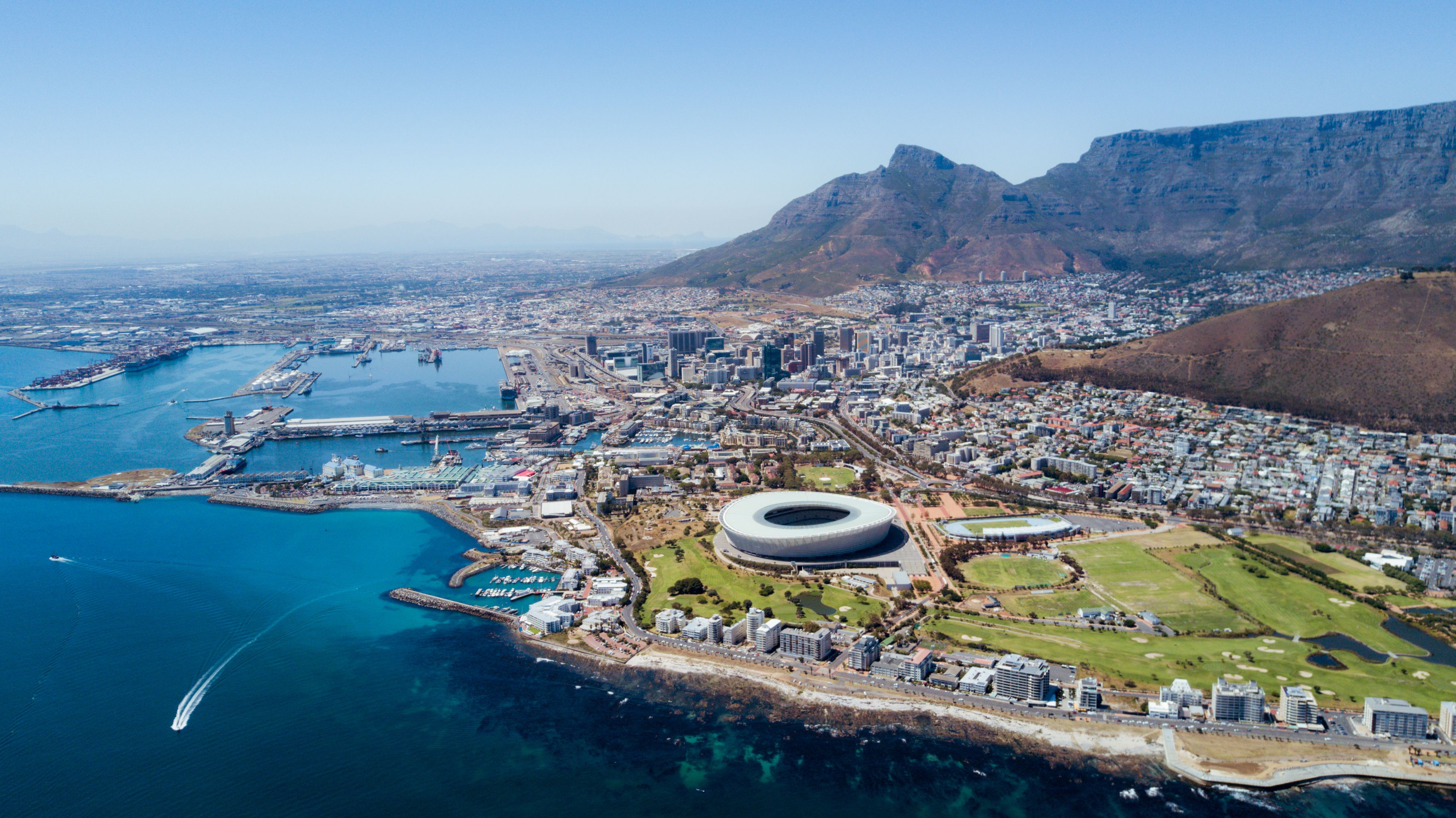
Prof Kasprzyk-Hordern’s group has been collaborating with researchers across the world, to use WBE for hazard forecasting and as an early warning system for global health risks.
Since 2017 she has worked on multiple UKRI Global Challenges Research Fund projects to implement early warning systems in Africa, in collaboration with Stellenbosch University in South Africa and the University of Lagos, Nigeria.
The most recent project tackled the problem of limited COVID-19 testing capacity in Africa by measuring the levels of RNA of SARS-Cov-2 in domestic wastewater.
The team is now developing a Digital Urban Environment Fingerprinting (DUEF) platform, a new approach in hazard forecasting and early-warning systems for global health risks and an extension to the existing concept of smart cities.
Prof Kasprzyk-Hordern says: “The COVID-19 pandemic has clearly highlighted the need for improved risk prediction in low resource settings, including urbanised regions in low to middle income countries where less physical distancing or access to rapid clinical testing makes it harder to mitigate disease spread in densely populated communities.
“Our DUEF platform looks at the complex mixture of substances in wastewater, including toxic chemicals, infectious microbes and human excretion products, anonymously pooled by the communities’ wastewater, which indicate the community-wide exposure to these compounds.
“We can then use this to look at environmental and community-wide exposure to chemicals and tally those with socioeconomic fingerprints."
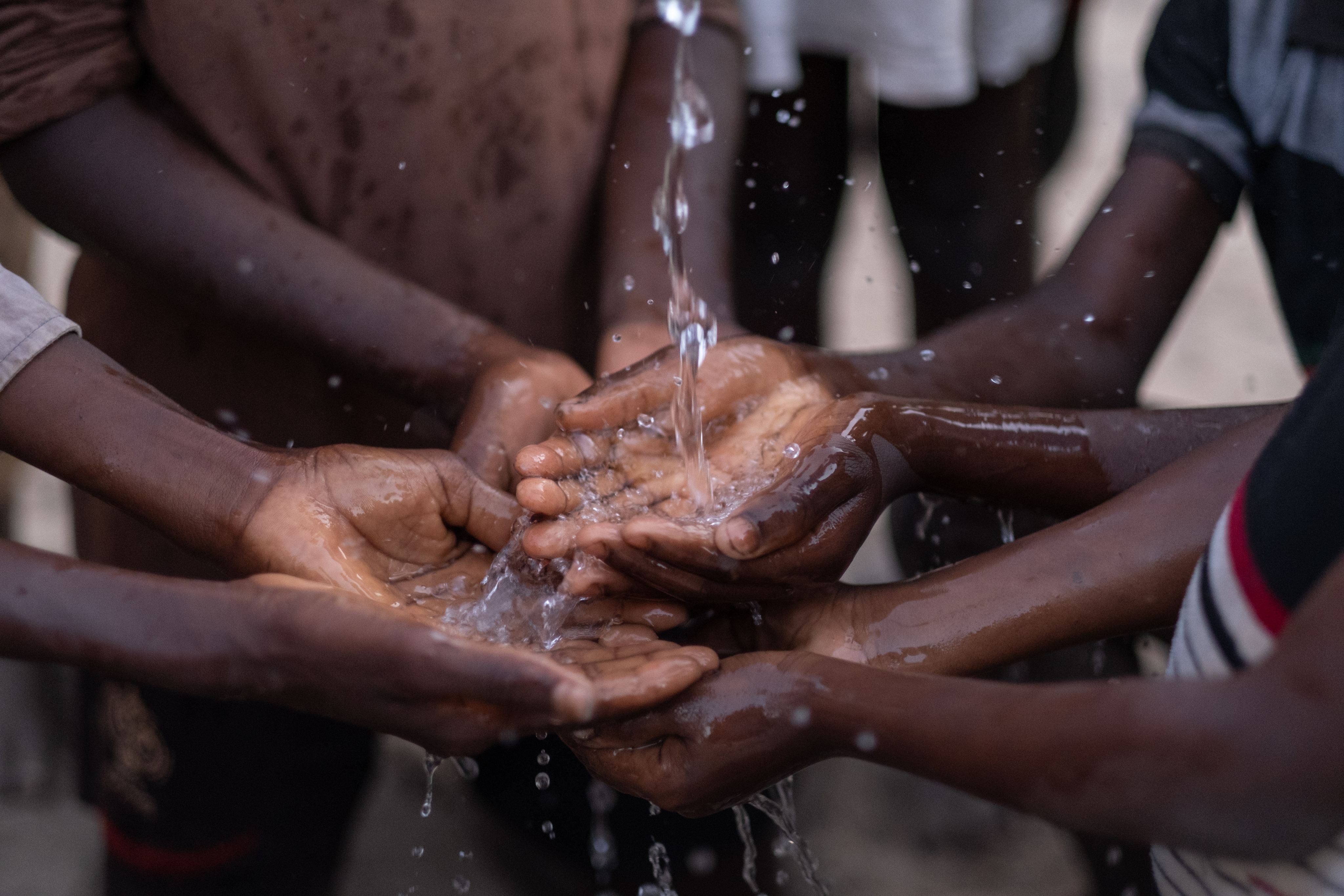
Photo by suheyp (Adobe Stock)
Photo by suheyp (Adobe Stock)
“We have two key aims: the first is simply making water cleaner for public and environmental health; and the next is providing an information platform to establish an early warning system for communities.
“Let’s say we had a baseline for a city and then the data showed a spike of pathogenic organisms; we could tell people to avoid the water before they get ill, rather than waiting for a local hospital to report a slew of cases once people have ingested it.
“We can also identify cities in need of new management strategies, including vulnerable communities, where there’s higher prevalence of drug use, or where industrial or other chemicals have been continuously or accidentally released into the environment exposing local populations too.
“We could also identify communities at higher risk of certain diseases linked to exposure to certain chemicals originating from local industrial or agricultural activities. This should in turn help with establishing management strategies by local authorities to minimise public health risks."
“We predict that in the future, these systems could be used as part of a One Health approach to safeguard both public and ecological health in urban areas in low to middle income countries.”
Stopping pollution at source
Closer to home, Prof Kasprzyk-Hordern’s team has also been working with Wessex Water to reduce the environmental levels of pharmaceuticals, using a new approach that could stop the drugs getting into the environment in the first place.
When a person swallows a medicine or other man-made substance, the ingested compounds are degraded by the body but not completely absorbed. The elements that aren’t broken down end up going through the sewage treatment process – this removes up to 90% of pharmaceuticals, however over time significant amounts still end up in rivers, lakes and soils.
This can have affects on the aquatic ecosystem, including fish, birds and microbes, as well as potentially eventually ending up in our drinking water.
In 2014, the EU Water Framework Directive was changed to legislate that, by 2018, certain pharmaceuticals in rivers must be reduced to extremely low levels to protect the natural environment and human health.
However, the higher level of wastewater treatment required to achieve this would be very expensive and inevitably lead to an increase in water bills.
Wessex Water estimated that it would cost around £2.2bn to reduce pharmaceuticals in wastewater discharges by 80% at wastewater treatment works in the Wessex Water region serving a population equivalent of 10,000 or more. The costs would be considerably higher for all water companies in England and Wales.
Prof Kasprzyk-Hordern says: “The current situation is unsustainable and needs disruptive change.
“What if, instead of treating the water after it had been polluted, we could prevent it happening in the first place by changing people’s behaviours?”
Prof Kasprzyk-Hordern joined forces with Professors Julie Barnett and Martyn Standage, both Professors of Psychology at the University of Bath’s Centre for Motivation and Health Behaviour Change, along with Ruth Barden, Richard Standerwick and Megan Robertson at Wessex Water.
Their study evaluating the impact of a population on water pollution found a direct link between the size of a city and the quantity of harmful chemicals and biological agents released naturally into the environment after passing through people’s bodies or directly discharged into the drains through activities such as showering and dish washing.
The five cities and towns used in the study (Bath, Bristol, Chippenham, Keynsham and Trowbridge) have different characteristics including population size, industry presence and socioeconomic status.
They found that the biggest offending polluters were painkillers and medications used for heart disease, mental-health conditions and epilepsy, along with antibiotics, lifestyle chemicals such as caffeine, and the substances produced when these compounds are broken down by the body.
Prof Barbara Kasprzyk-Hordern said: “What is clear from this study is that our lifestyle choices – linked with the household and beauty products we use, and the pharmaceuticals we consume, have a direct impact on the surrounding environment.
“Most of us are unaware of this impact because each use of a product results in small parts-per-billion quantities of toxic waste that can’t be seen with a naked eye, but when taken together, these tiny quantities create a complex chemical cocktail in our rivers that might have detrimental effects on the wider environment, especially aquatic creatures.”
The researchers propose that instead of employing energy-intensive, expensive treatment works, social interventions that target pharmaceutical usage could greatly reduce the pollution at source.
These measures include:
- Awareness campaigns to return unused medications to pharmacies instead of disposing them in the bin or down the toilet
- Green prescribing, where the medication with the least environmental impact is prescribed where there is a choice of drugs for a condition
- Social prescribing, where medicines are prescribed as a last resort and instead doctors prescribe non-pharmaceutical treatments or activities, such as dietary, exercise and wellbeing support.
Prof Julie Barnett says: “There is considerable scope for changes in prescribing practices to have an impact on environmental health. Which pharmaceuticals are prescribed or de-prescribed, whether a social prescription may be appropriate and how we dispose of unused pharmaceuticals are all areas where changes can make important differences to the health of the wider environment.”
The work by Kasprzyk-Hordern’s group has led to the widespread interest in application of water fingerprinting policies and practices by Wessex Water, DEFRA, the Environment Agency and local authorities.
The longstanding collaboration with the University of Bath has enabled Wessex Water to put into practice the holistic approach that tackles disposal of pharmaceuticals at source.
The multiple projects coordinated by Prof Kasprzyk-Hordern have allowed considerable relationship-building with new organisations working in and around the South West region, the Environment Agency, Bath & North East Somerset Public Health and Defra as well as the Royal United Hospital (RUH) in Bath and NHS England and organisations undertaking social prescribing and wellbeing activities.
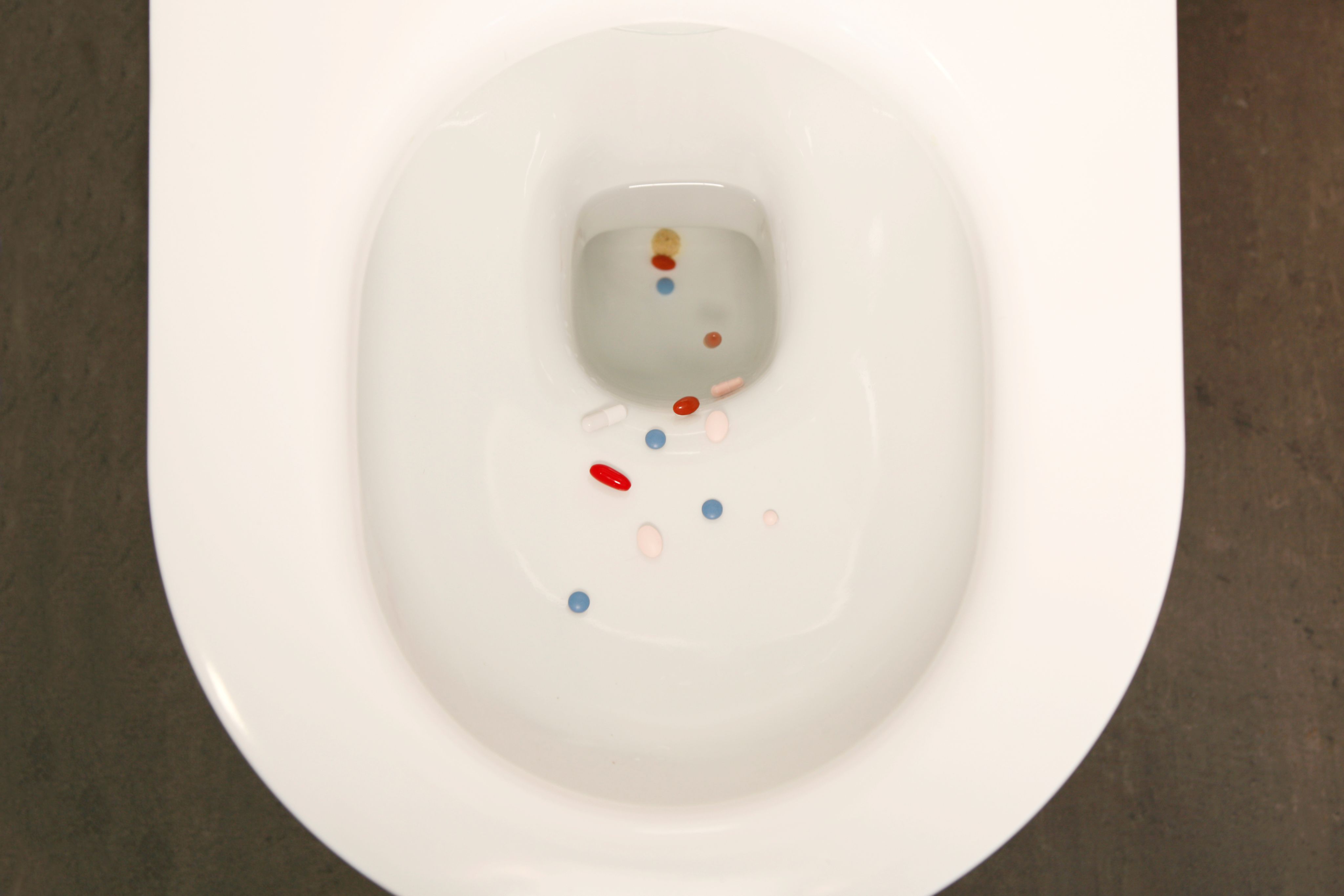
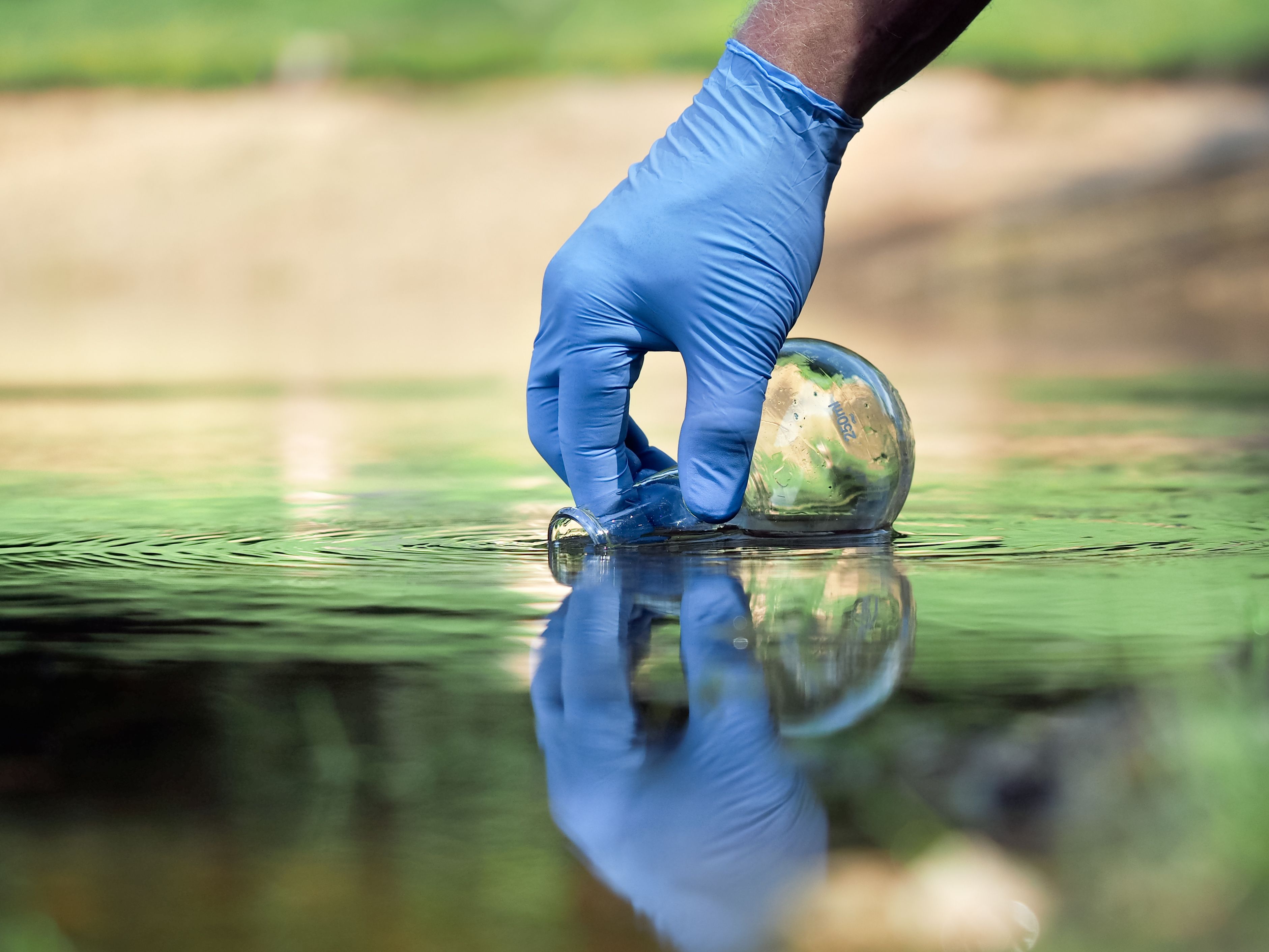
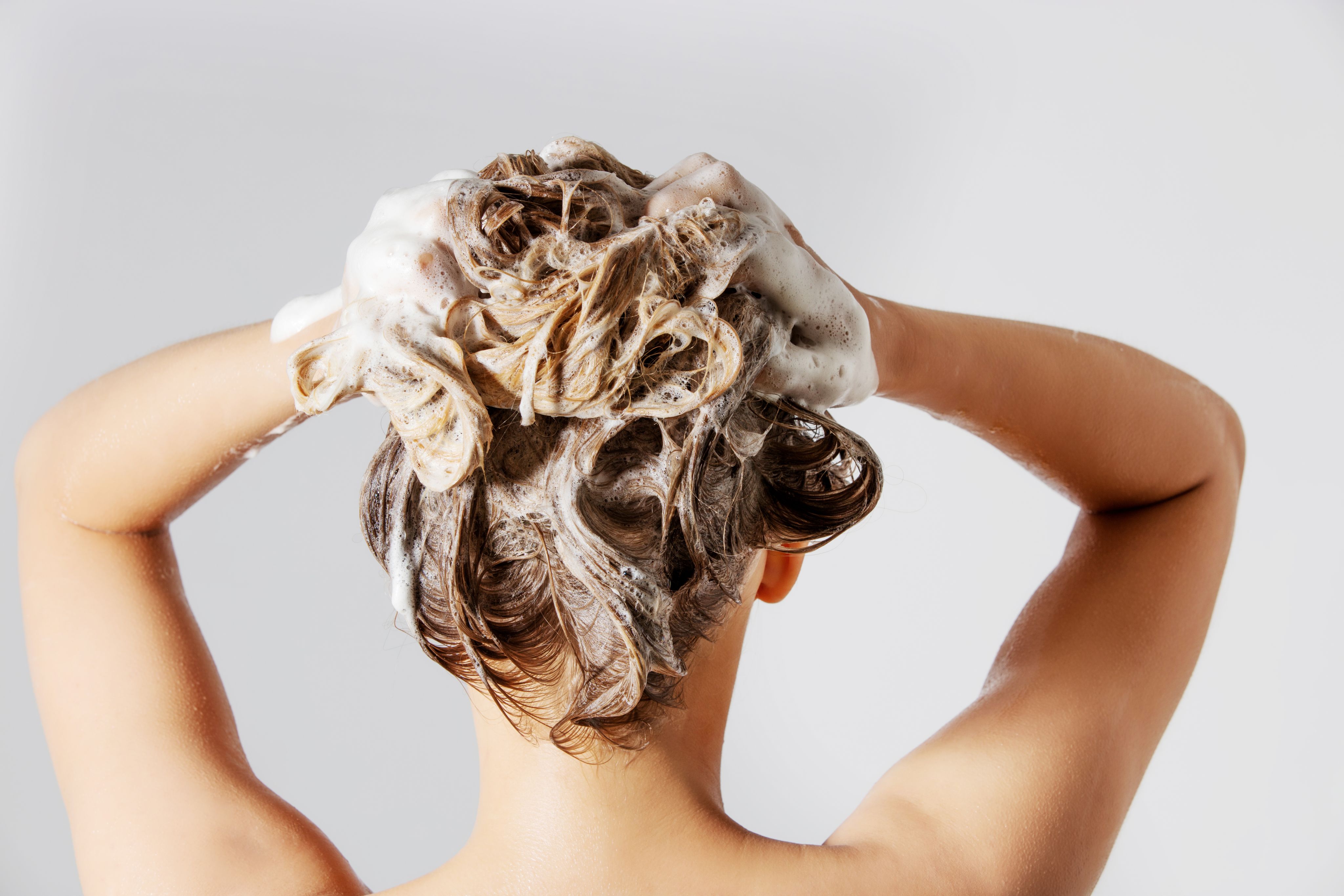
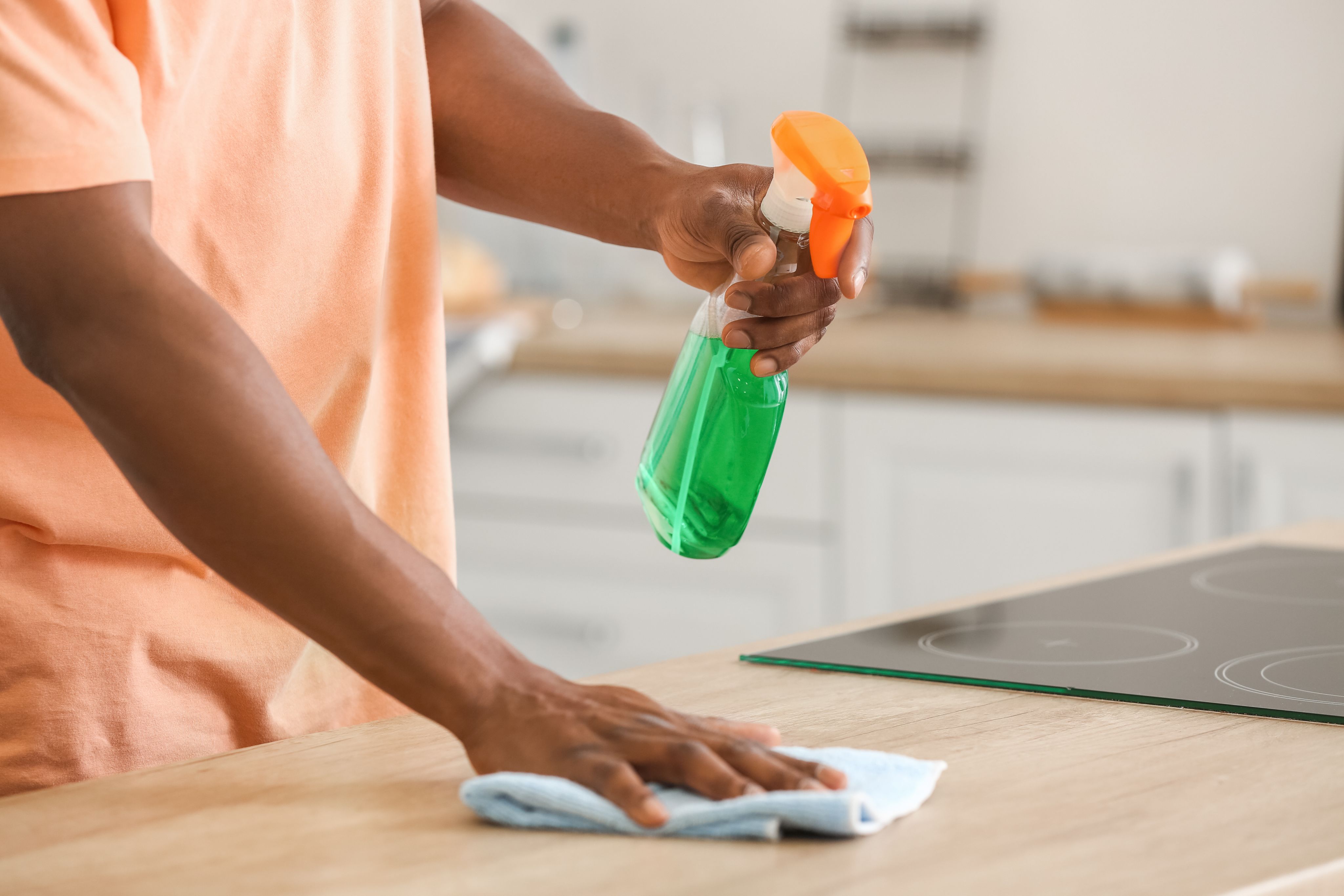
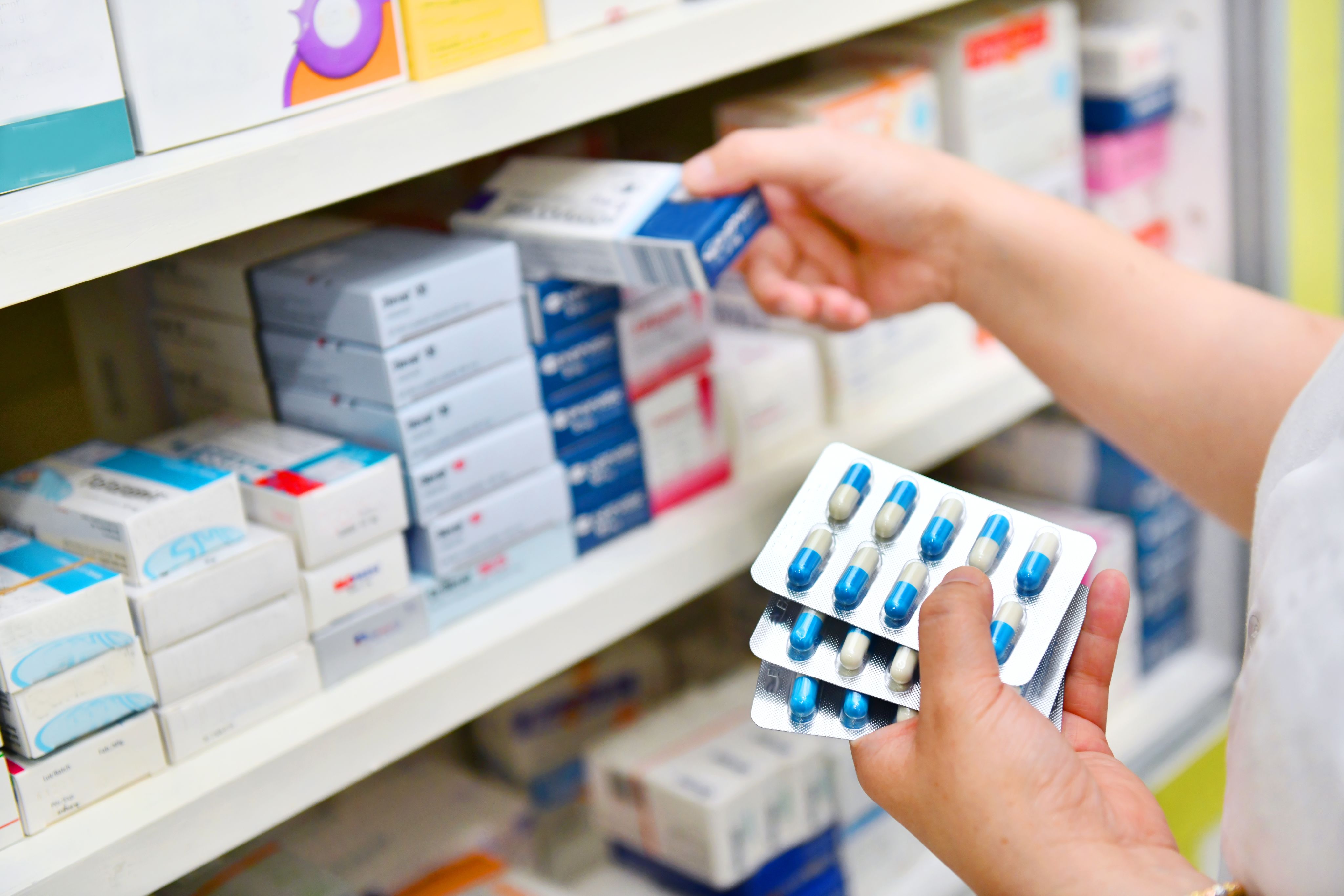
Closer to home, Prof Kasprzyk-Hordern’s team has also been working with Wessex Water to reduce the environmental levels of pharmaceuticals, using a new approach that could stop the drugs getting into the environment in the first place.
When a person swallows a medicine or other man-made substance, the ingested compounds are degraded by the body but not completely absorbed. The elements that aren’t broken down end up going through the sewage treatment process – this removes up to 90% of pharmaceuticals, however over time significant amounts still end up in rivers, lakes and soils.
This can have affects on the aquatic ecosystem, including fish, birds and microbes, as well as potentially eventually ending up in our drinking water.
In 2014, the EU Water Framework Directive was changed to legislate that, by 2018, certain pharmaceuticals in rivers must be reduced to extremely low levels to protect the natural environment and human health.
However, the higher level of wastewater treatment required to achieve this would be very expensive and inevitably lead to an increase in water bills.
Wessex Water estimated that it would cost around £2.2bn to reduce pharmaceuticals in wastewater discharges by 80% at wastewater treatment works in the Wessex Water region serving a population equivalent of 10,000 or more. The costs would be considerably higher for all water companies in England and Wales.
Prof Kasprzyk-Hordern says: “The current situation is unsustainable and needs disruptive change.
“What if, instead of treating the water after it had been polluted, we could prevent it happening in the first place by changing people’s behaviours?”
Prof Kasprzyk-Hordern joined forces with Professors Julie Barnett and Martyn Standage, both Professors of Psychology at the University of Bath’s Centre for Motivation and Health Behaviour Change, along with Ruth Barden, Richard Standerwick and Megan Robertson at Wessex Water.
Their study evaluating the impact of a population on water pollution found a direct link between the size of a city and the quantity of harmful chemicals and biological agents released naturally into the environment after passing through people’s bodies or directly discharged into the drains through activities such as showering and dish washing.
The five cities and towns used in the study (Bath, Bristol, Chippenham, Keynsham and Trowbridge) have different characteristics including population size, industry presence and socioeconomic status.
They found that the biggest offending polluters were painkillers and medications used for heart disease, mental-health conditions and epilepsy, along with antibiotics, lifestyle chemicals such as caffeine, and the substances produced when these compounds are broken down by the body.
Prof Barbara Kasprzyk-Hordern said: “What is clear from this study is that our lifestyle choices – linked with the household and beauty products we use, and the pharmaceuticals we consume, have a direct impact on the surrounding environment.
“Most of us are unaware of this impact because each use of a product results in small parts-per-billion quantities of toxic waste that can’t be seen with a naked eye, but when taken together, these tiny quantities create a complex chemical cocktail in our rivers that might have detrimental effects on the wider environment, especially aquatic creatures.”
The researchers propose that instead of employing energy-intensive, expensive treatment works, social interventions that target pharmaceutical usage could greatly reduce the pollution at source.
These measures include:
- Awareness campaigns to return unused medications to pharmacies instead of disposing them in the bin or down the toilet
- Green prescribing, where the medication with the least environmental impact is prescribed where there is a choice of drugs for a condition
- Social prescribing, where medicines are prescribed as a last resort and instead doctors prescribe non-pharmaceutical treatments or activities, such as dietary, exercise and wellbeing support.
Prof Julie Barnett says: “There is considerable scope for changes in prescribing practices to have an impact on environmental health. Which pharmaceuticals are prescribed or de-prescribed, whether a social prescription may be appropriate and how we dispose of unused pharmaceuticals are all areas where changes can make important differences to the health of the wider environment.”
The work by Kasprzyk-Hordern’s group has led to the widespread interest in application of water fingerprinting policies and practices by Wessex Water, DEFRA, the Environment Agency and local authorities.
The longstanding collaboration with the University of Bath has enabled Wessex Water to put into practice the holistic approach that tackles disposal of pharmaceuticals at source.
The multiple projects coordinated by Prof Kasprzyk-Hordern have allowed considerable relationship-building with new organisations working in and around the South West region, the Environment Agency, Bath & North East Somerset Public Health and Defra as well as the Royal United Hospital (RUH) in Bath and NHS England and organisations undertaking social prescribing and wellbeing activities.
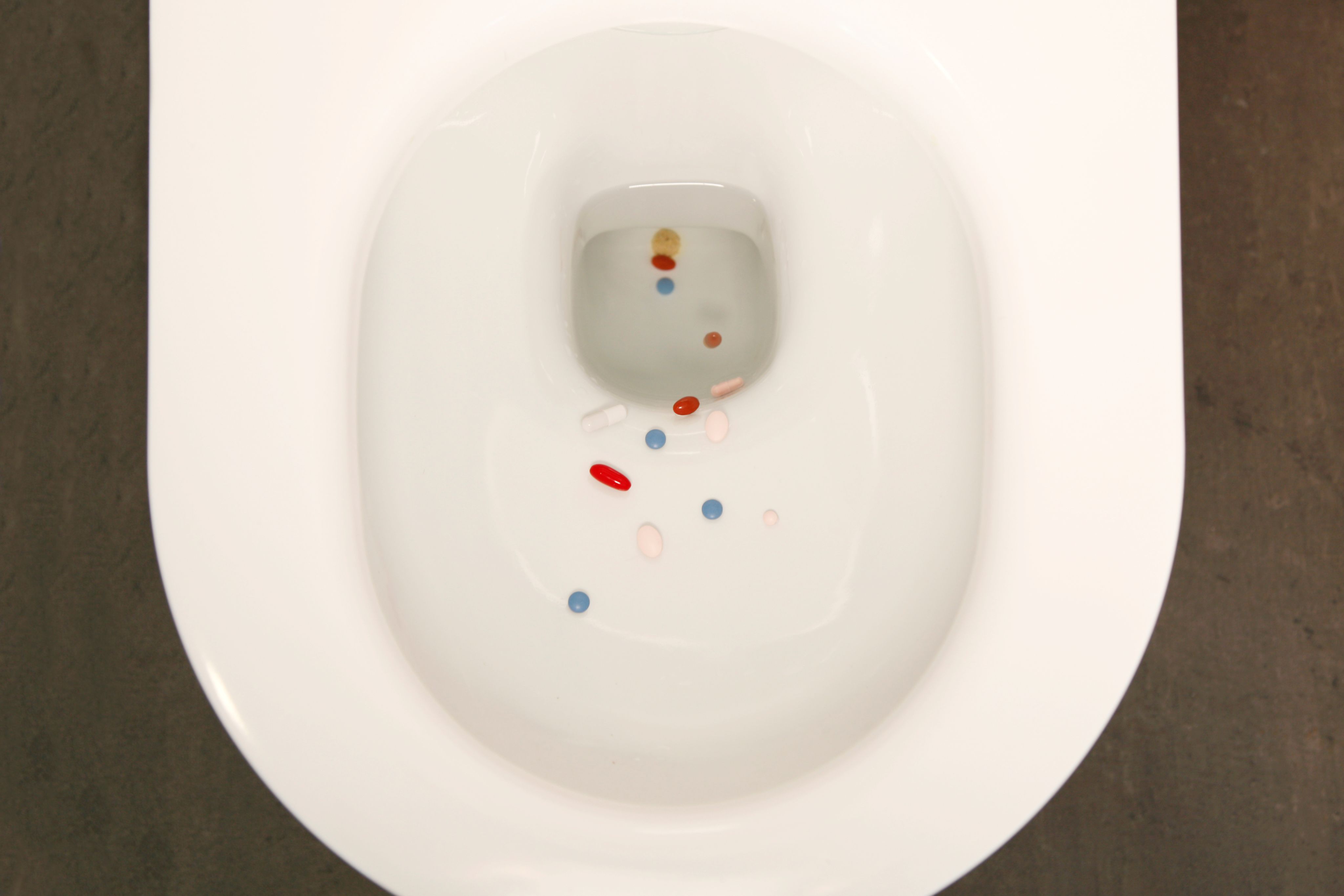
Photo by ImagESine (Adobe Stock)
Photo by ImagESine (Adobe Stock)
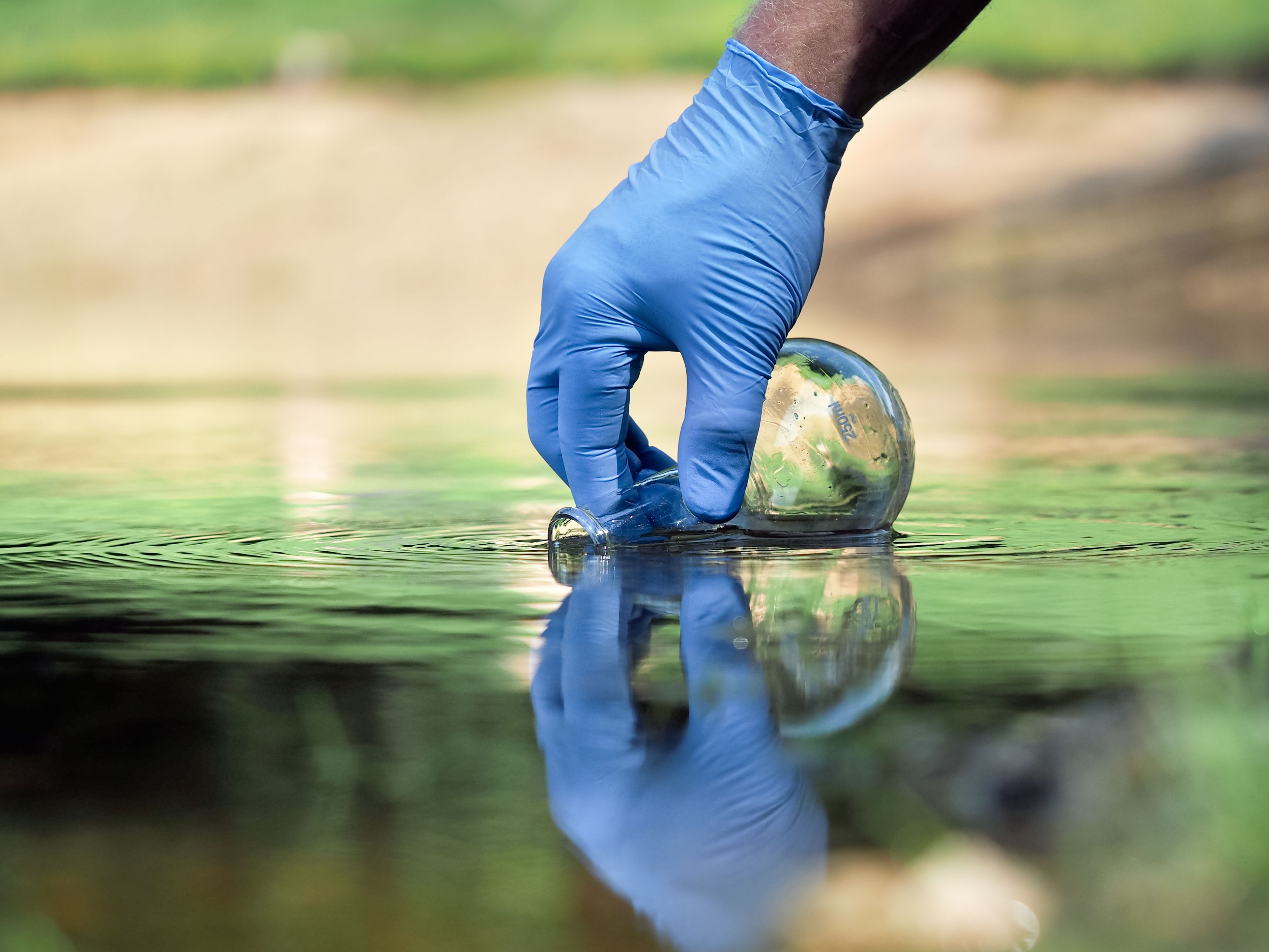
Photo by kozorog (Adobe Stock)
Photo by kozorog (Adobe Stock)
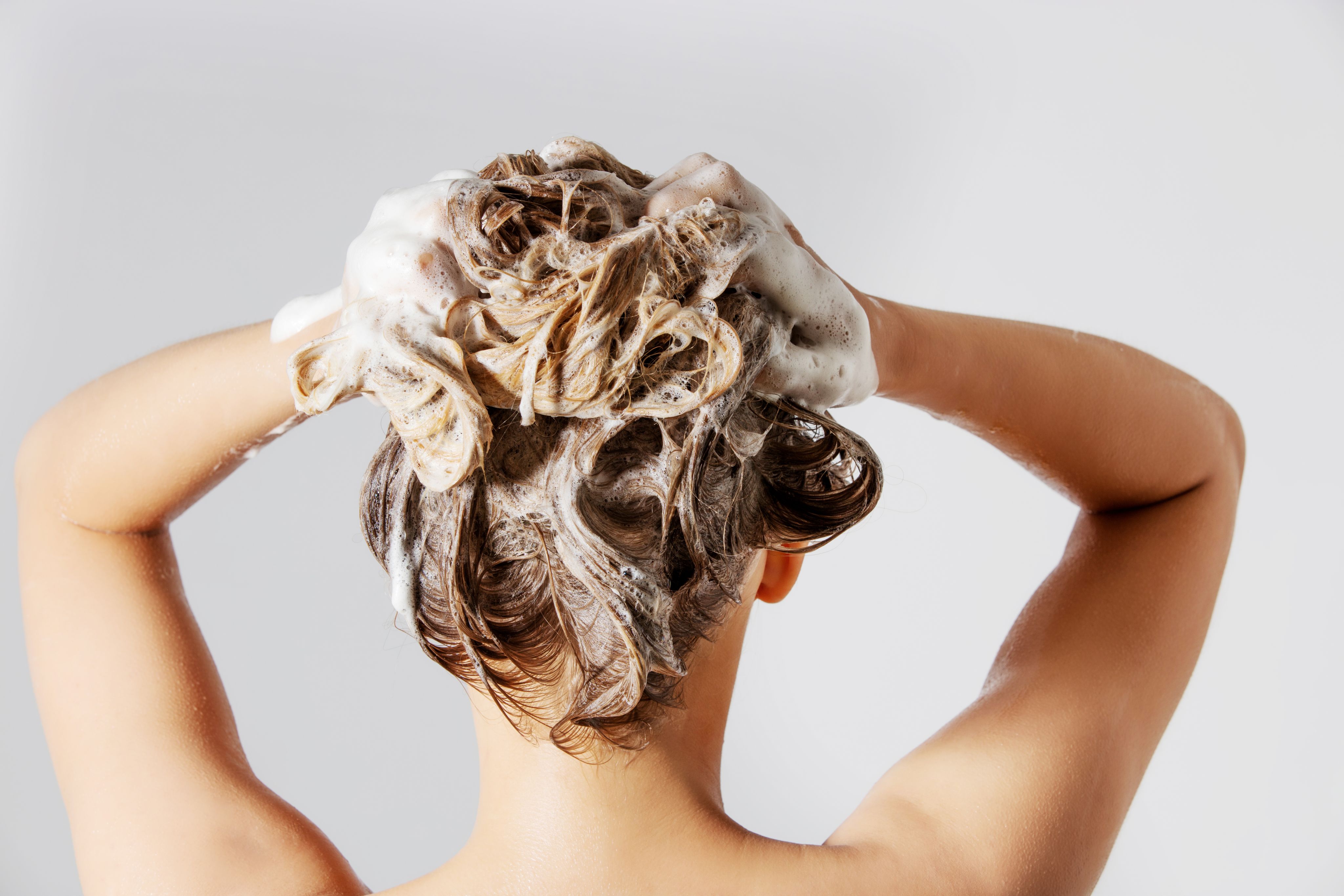
Photo by Piotr Marcinski (Adobe Stock)
Photo by Piotr Marcinski (Adobe Stock)
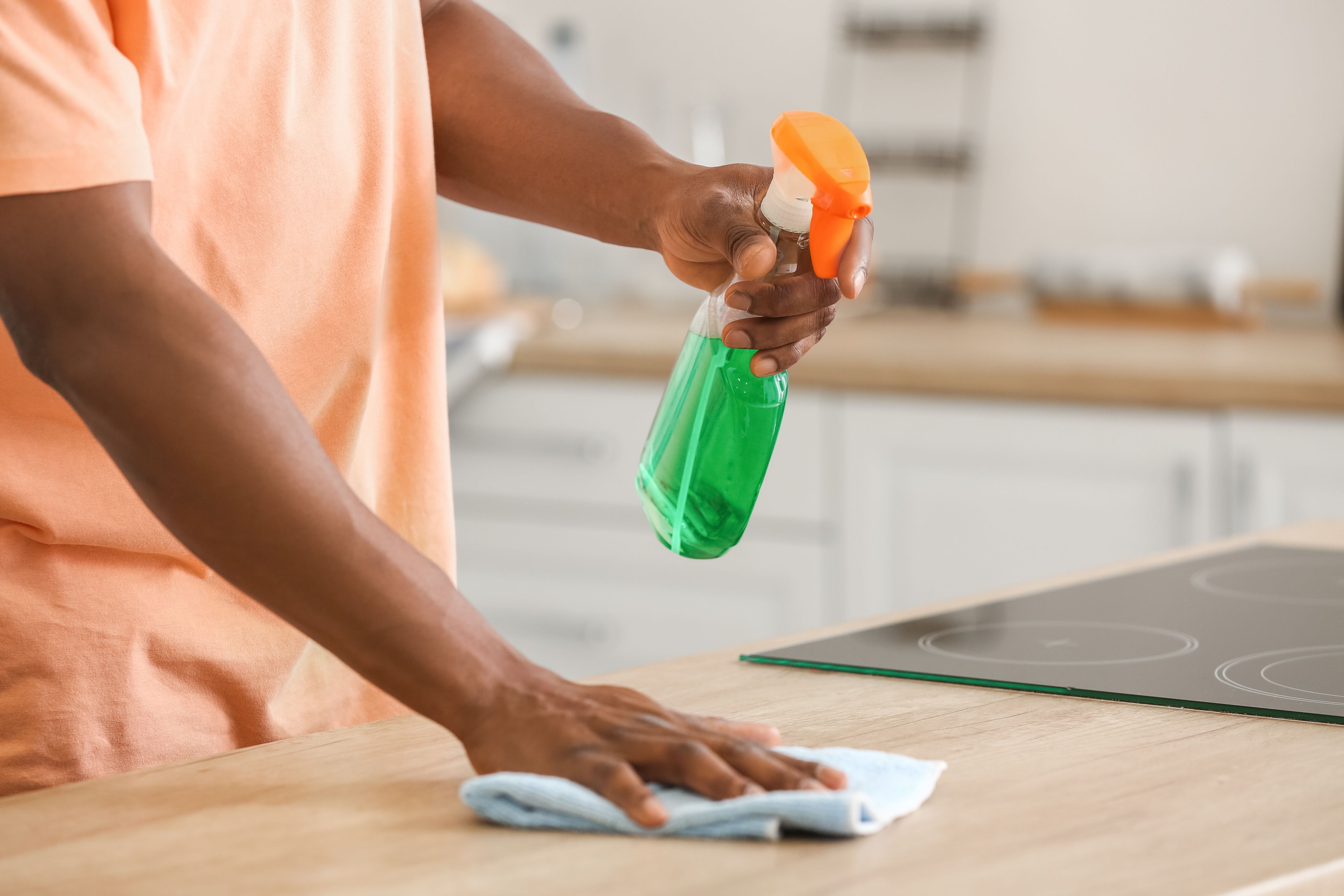
Adobe Stock
Adobe Stock
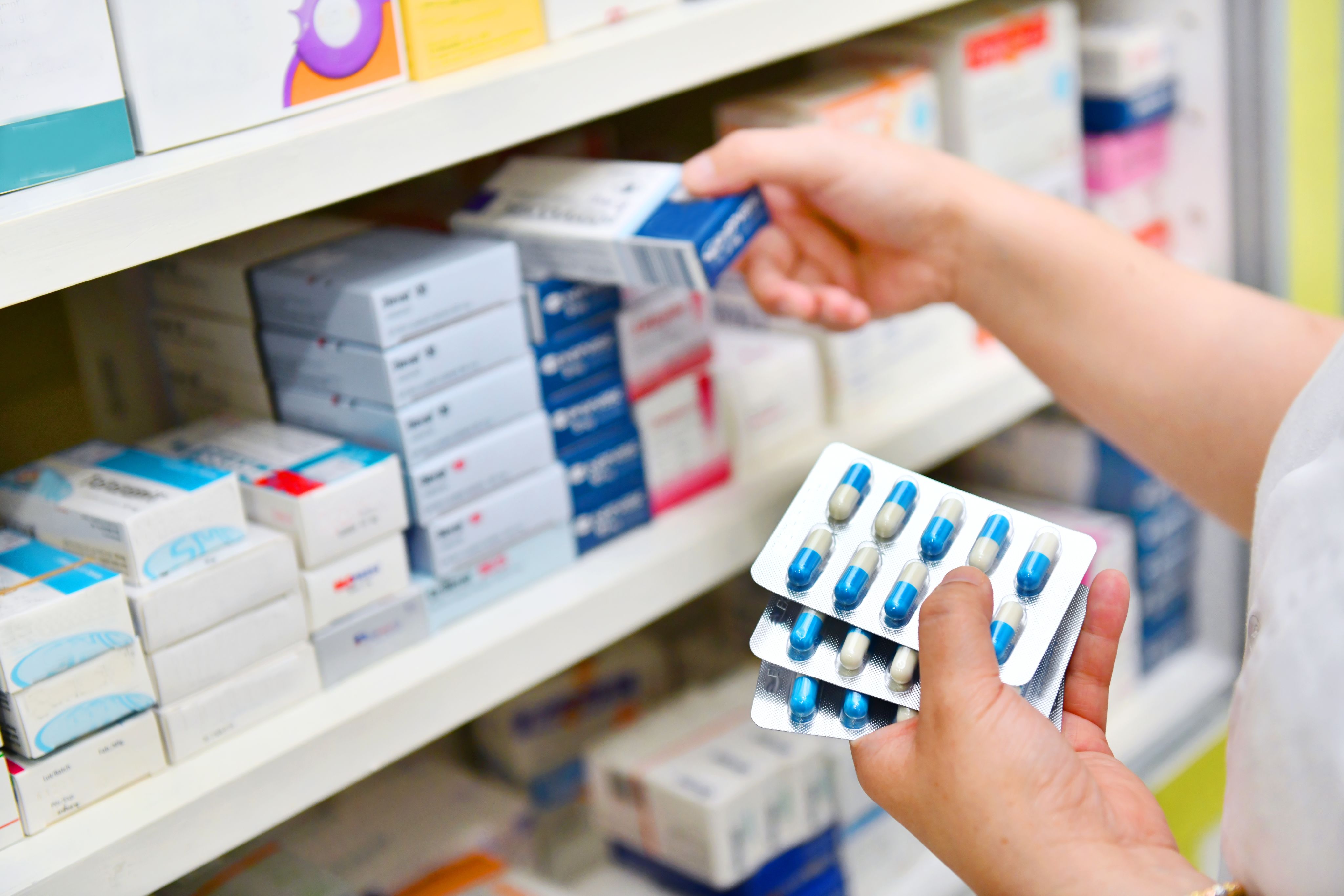
Photo by Viewfinder (Adobe Stock)
Photo by Viewfinder (Adobe Stock)
Limitations and challenges
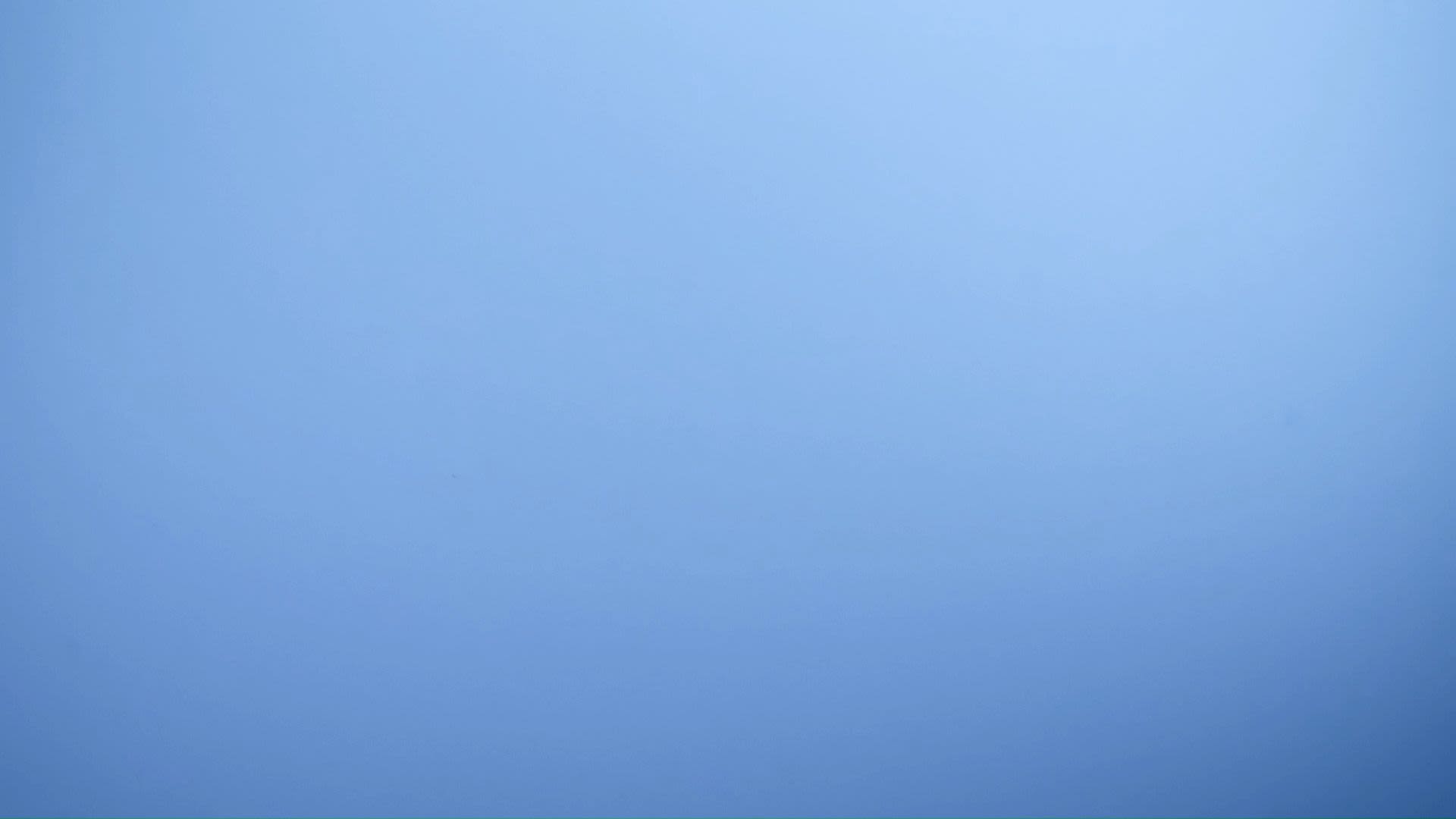
Whilst it’s a powerful tool, WBE does have its limitations.
The accuracy of analysis depends on where sample is taken, how long it’s travelled from its source, whether the water has been treated and whether the chemicals being tracked have degraded on their journey.
Also, it can be difficult to accurately quantify the number of people covered by the sample.
Plus, there are ethical concerns when using wastewater surveillance to track people’s lifestyles, if samples are taken near the source rather than at wastewater treatment plants.
Prof Kasprzyk-Hordern says: “Although samples are pooled and anonymous, if samples are taken very close to the source, there could be concerns of stigmatising particular community groups.
“We need to develop guidelines and a social license to operate if the approach is to be successfully adopted widely.”
Next steps
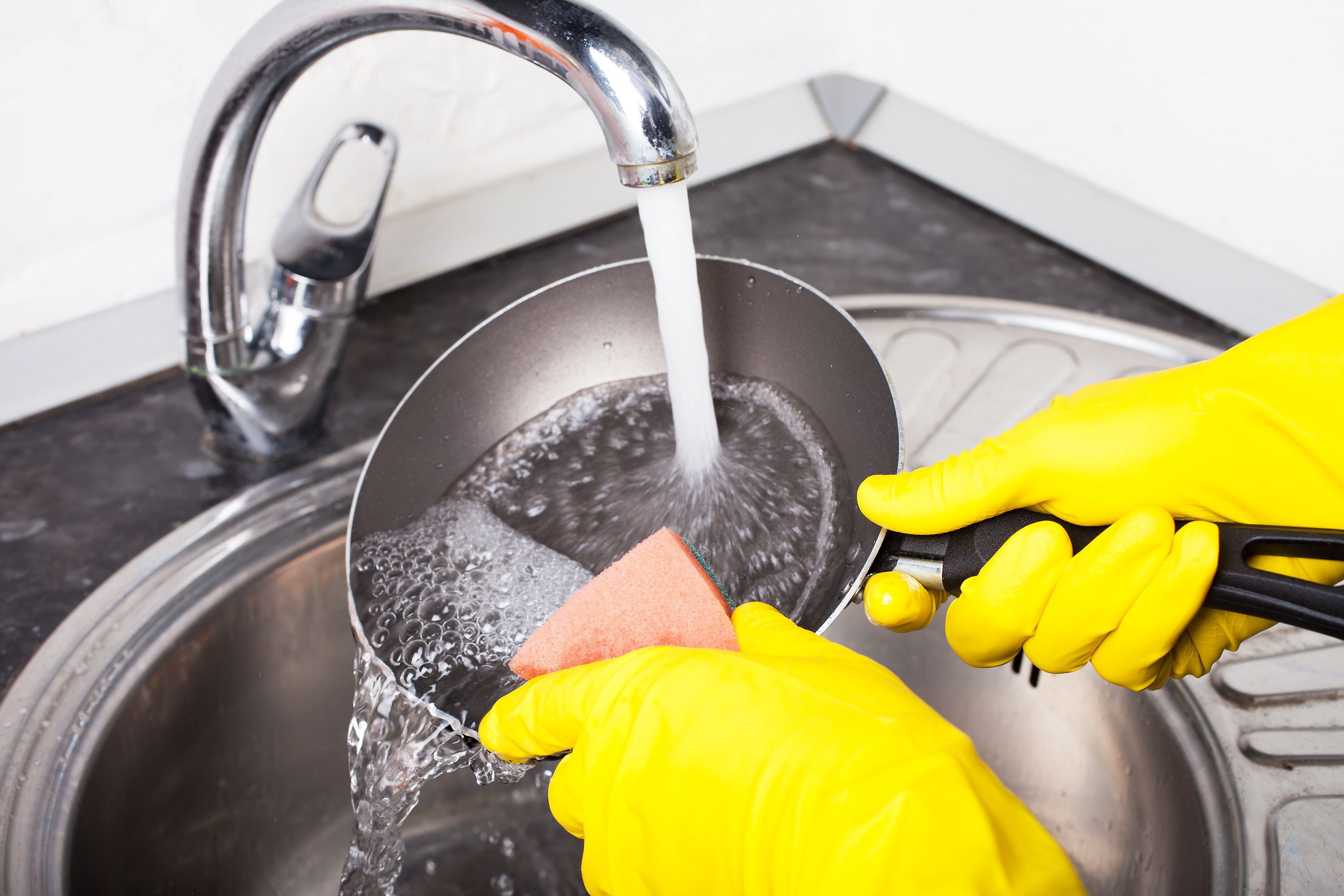
Prof Kasprzyk-Hordern’s research has diversified into looking at other chemicals and their effects on the environment and our health.
Having already used WBE to quantify the usage of antibiotics in the community, she’s working with colleague Prof Edward Feil in the University of Bath’s Milner Centre for Evolution to develop methods of tracking the spread of antibiotic resistance, by detecting the bacterial genes associated with resistance.
Her team is also developing the chemical techniques to detect endocrine disruptors - chemicals found in everything from personal care products like cosmetics and lotions to general household cleaning products - which have been shown to mimic or interfere with the body’s hormones, known as the endocrine system.
She is also looking at so-called “forever chemicals” including BPA (found in some plastics) and perfluorinated chemicals, found in the non-stick coatings of frying pans, amongst other things.
Prof Kasprzyk-Hordern says: “People assume that because they aren’t ingested, these chemicals don’t end up in the body, but in reality they get into the water system and so traces end up in our food chain or drinking water.
“They can also directly leach from plastic food packaging and other materials to food or when applied directly to skin.”
“These chemicals have been loosely linked with conditions like infertility and diabetes, but if we can measure the exposure of people to these, and determine whether they have been metabolised by the body, we can do longitudinal studies to correlate them with socioeconomic geography and disease incidence."
Prof Kasprzyk-Hordern has recently started co-leading a project with Prof Werner Brack from UFZ Helmholtz Centre for Environmental Research (Germany) to do just this as part of a €400 million major research project, PARC.
This is a seven-year collaboration between 200 partners in 28 countries and will focus on developing new epidemiology tools to assess public exposure to hazardous chemicals and assess the associated risks using data collected from wastewater treatment plants throughout Europe.
The results of the programme will be used to support new European and national strategies to reduce exposure to hazardous chemicals and their impact on health and the environment.
“WBE is an incredibly powerful tool with the potential to provide the real-world evidence for any links of these chemicals to disease.”
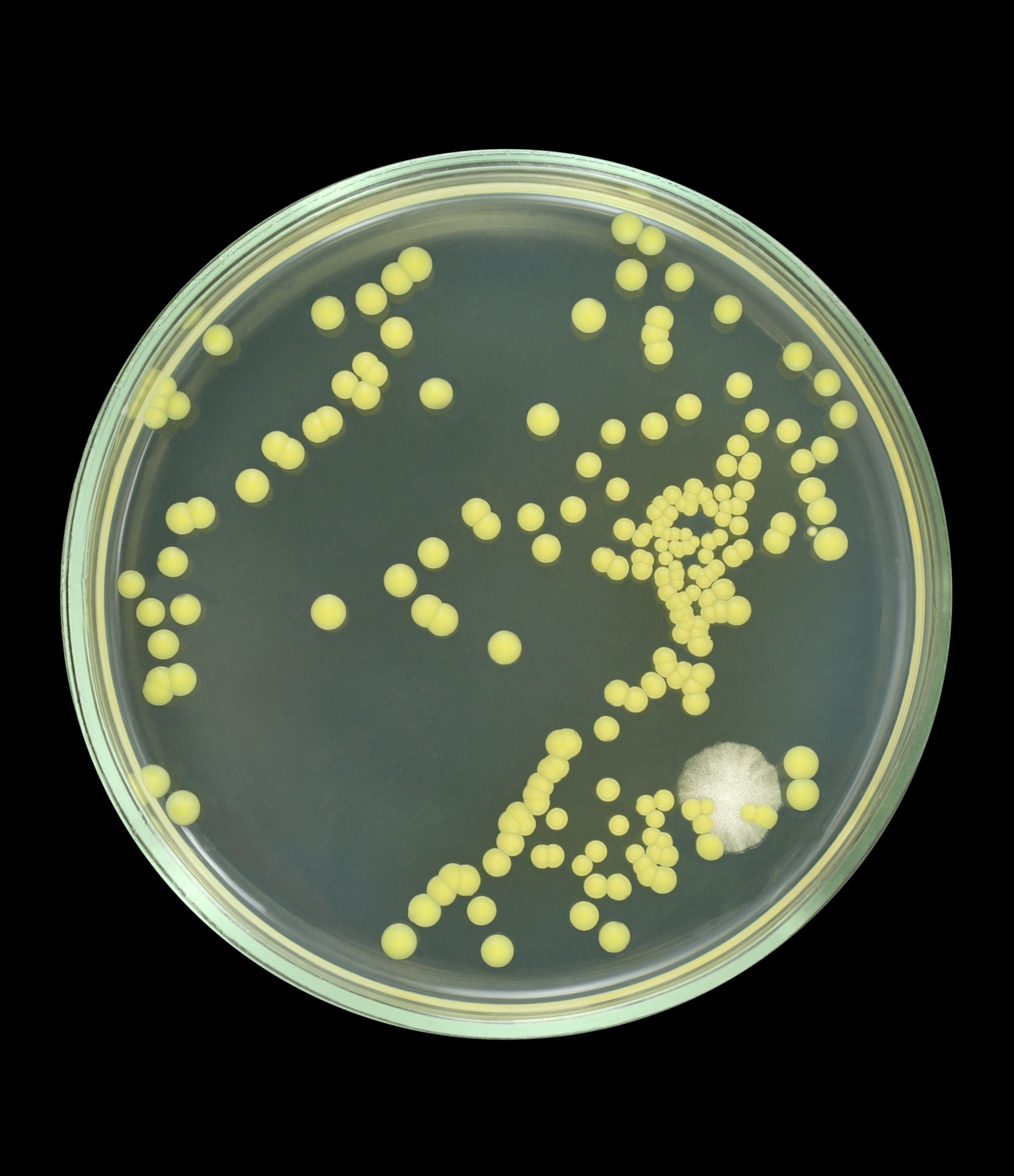
WBE is being used to track the spread of antibiotic resistance. (Photo by Satirus on Adobe Stock)
WBE is being used to track the spread of antibiotic resistance. (Photo by Satirus on Adobe Stock)
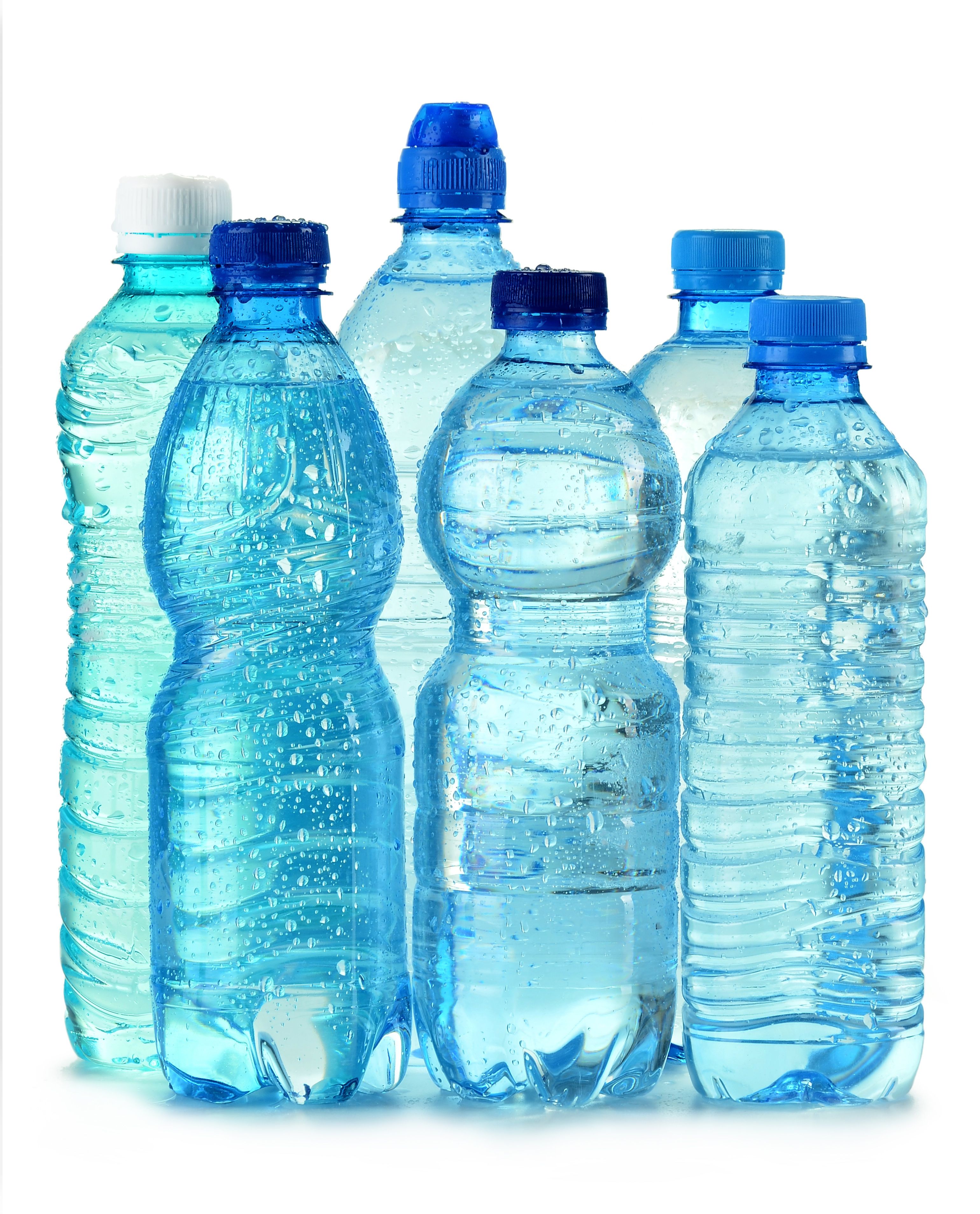
Chemicals such as BPA in plastic packaging can leach into food or water (Photo by monticellllo on Adobe Stock)
Chemicals such as BPA in plastic packaging can leach into food or water (Photo by monticellllo on Adobe Stock)
Research papers
Wastewater-based epidemiology in hazard forecasting and early-warning systems for global health risks
Barbara Kasprzyk-Hordern et al. (2022) Environment International https://doi.org/10.1016/j.envint.2022.107143
Human population as a key driver of biochemical burden in an inter-city system: Implications for One Health concept
Barbara Kasprzyk-Hordern, Kathryn Proctor, Kishore Jagadeesan, Felicity Edler, Richard Standerwick, Ruth Barden (2022) Journal of Hazardous Materials 429:127882 https://doi.org/10.1016/j.jhazmat.2021.127882
Spatio-temporal assessment of illicit drug use at large scale: evidence from 7 years of international wastewater monitoring
González-Mariño, I. et al, 26 Dec 2019, In: Addiction. 115, 1, p. 109-120 12 p.
Spatiotemporal profiling of antibiotics and resistance genes in a river catchment: Human population as the main driver of antibiotic and antibiotic resistance gene presence in the environment
Felicity CT Elder, Kathryn Proctor, Ruth Barden, William H Gaze, Jason Snape, Edward J Feil, Barbara Kasprzyk-Hordern (2021) Water Research
Estimation of community-wide multi-chemical exposure via water-based chemical mining: key research gaps drawn from a comprehensive multi-biomarker multi-city dataset
Barbara Kasprzyk-Hordern, Kathryn Proctor, Kishore Jagadeesan, Luigi Lopardo, Kieran J O'Daly, Richard Standerwick, Ruth Barden (2021) Environment International https://doi.org/10.1016/j.envint.2020.106331
Challenges in realising the potential of wastewater-based epidemiology to quantitatively monitor and predict the spread of disease
Julian Faraway, James Boxall-Clasby, Edward J Feil, Marjorie J Gibbon, Oliver Hatfield, Barbara Kasprzyk-Hordern, Theresa Smith (2022) Journal of Water and Health https://doi.org/10.2166/wh.2022.020
Diagnosing Down-the-Drain Disposal of Unused Pharmaceuticals at a River Catchment Level: Unrecognized Sources of Environmental Contamination That Require Nontechnological Solutions
Barbara Kasprzyk-Hordern, Kathryn Proctor, Kishore Jagadeesan, Scott Watkins, Richard Standerwick, Ruth Barden, Julie Barnett (2021) Environmental Science & Technology https://doi.org/10.1021/acs.est.1c01274
Wastewater-Based Epidemiology for the assessment of population exposure to chemicals: the need for integration with Human Biomonitoring for global One Health actions
Barbara Kasprzyk-Hordern, Frederic Béen, Lubertus Bijlsma, Werner Brack, Sara Castiglioni, Adrian Covaci, Bice S. Martincigh, Jochen F. Mueller, Alexander L.N. van Nuijs, Temilola Oluseyi, Kevin V. Thomas (2023) Journal of Hazardous Materials https://doi.org/10.1016/j.jhazmat.2023.131009
Photos by Laurie Lapworth unless otherwise credited.
Video by Simon Wharf.
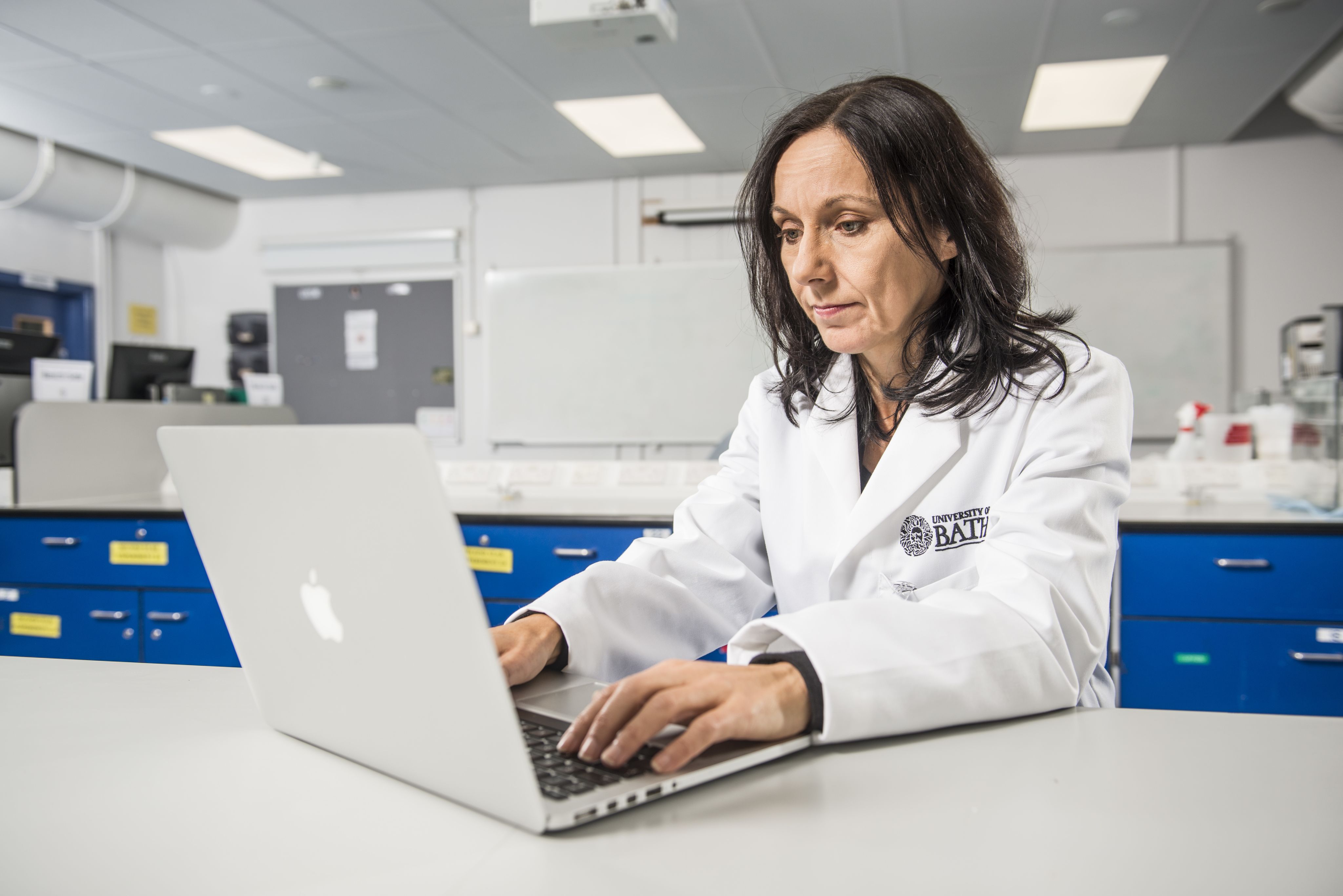